> Note |
Warped PassagesUnravelling the mysteries of the universe's hidden dimensions
|
Cat: SCO |
Lisa Randall |
16605u/18227r |
Original resume |
Remarks |
>Top0. Preface:
|
0. 序文:
|
>Top 1. Entryway Passage:
|
1. 入り口の通路:
|
>Top 2. Restricted Passage: Rolled-up Extra Dimensions
|
2. 限定的な通路: 巻き上げられた余剰次元
|
>Top 3. Exclusive Passage: Branes, Braneworlds, and the Bulk
|
3. 排他的な通路: ブレイン、ブレイン世界、バルク:
|
>Top 4. Approaches to Theoretical Physics:
|
4. 理論物理学へのアプローチ:
|
|||||||||||||||||||||||||
|
>Top 5. Relativity; The Evolution of Einstein's Gravity:
|
5. 相対論: アインシュタインの重力の進化
|
>Top 6. Quantum Mechanics: Principled uncertainty, Principal uncertainties, and the uncertainty principle:
|
6. 量子力学: 不確定問題
|
>Top 7. The Standard Model of Particle Physics: Matter's most basic known structure:
|
7. 素粒子物理学の標準モデル:
|
>Top | Quarks: experience strong force | Leptons | |||||
1st generation (stable) |
Up-L 3 MeV |
Down-L 7 MeV |
Up-R 3 MeV |
Down-R 7 MeV |
$e$ neutrino-L 〜0 |
$e$-L 0.5 MeV |
$e$-R 0.5 MeV |
2nd generation | Charm-L 1.2 GeV |
Strange-L 120 MeV |
Charm-R 1.2 GeV | Strange-R 120 MeV |
$\mu$ neutrino-L 〜0 |
$\mu$-L 106 MeV |
$\mu$-R 106 MeV |
3rd gneration | Top-L 174 GeV |
Bottom-L 4.3 GeV |
Top-R 174 GeV |
Bottom-R 4.3 GeV |
$\tau$ neutrino-L 〜0 |
$\tau$-L 1.8 GeV |
$\tau$-R 1.8 GeV |
Quarks-L: weak force | Leptons-L: weak force |
|
>Top 8. Experimental Interlude; Verifying the Standard Model:
- High-energy physics; high-energy particle collider
- Experiments at high-energy colliders will tell us about fundamental laws of nature that cannot be observed in any other way.
- Top Quark Discovery:
- the Standard Model would be inconsistent without it; as recently as 1995, no one had ever detected one.
- Bottom quark, which weights at 5 times the mass of a proton, was discovered in 1977.
- Top quark was remarkably heavy compared with the other quarks.
- Finally appeared after 20 years of searching; there were trillion collision events that didn't contain a top quark.
- Tevatron (<TeV) located at Fermilab, in Batavia, Illinois, 50 km west of Chicago;
- Many heavy particles are unstable and decay almost immediately.
- Experiments search for visible evidence of a particle's decay products, rather than the particle itself.
- CERN (Conseil Européen pur la Recherche Nucléaire) in Switzerland:
- Tim Berners-Lee; came up with WWW and HTML.
- LHC (Large Hadron Collider) will be built within a decade; will be able to reach 7 times the present energy of the Tevatron.
- To determine the precise value of Z bozon's mass; they had to take into account everything that might affect the value of its energy.
- Tides in Lake Geneva; which sightly altered the distance over which the electrons and positrons traveled inside the collider
- TGV, the express train that ravels between Geneva and Paris; power spikes associated with the French DC current that slightly disrupted the acceleration; there was inevitably a strike, treating to a spike-free day!
8. 実験的な幕間:
- the Standard Model:
-
Fermions
half integer spin
Qaurks
U, D, C, S, T, B (quark/antiquark)
Leptons
Electron, Positron,
Muon, Antimuon
Tau, Antitau
Electron neutrino, Electron antineutrino
Muon neutrino, Muon antineutrino
Tau neutrino,
Tau antineutrino
Bosons
integer spin
Gauge
Photon, Gluon, W & Z bosons
Scalar
Higgs boson
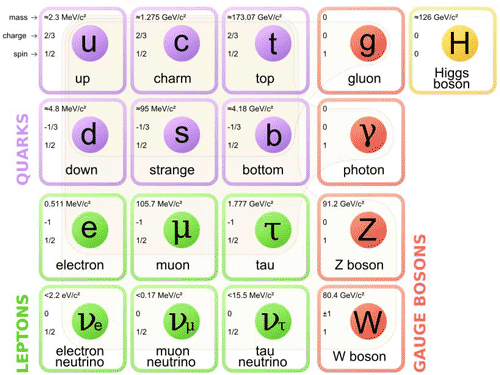
>Top 9. Symmetry:
- the Standard Model:
- is a theory of quarks, leptons, and weak gauge bosons (Ws and Z); all have mass.
- if matter had been truly massless, it wouldn't form nice, solid objects, and structure and life in the universe.
- Symmetry:
- is a sacred word to most physicists.
- Christian cross, Jewish menorah, Dharma wheel of Buddhism, Crescent of Islam, Hindu mandala.
- Symmetry can be found in most paintings sculpture, architecture, music, dance, and poetry; Islamic art, Taj Mahal
- Symmetry is a natural player; when a physical system has symmetry, you can describe the system on the basis of fewer observations than if the system had no symmetry.
- if a system possesses rotational and translational symmetries, physical laws apply the same way in all directions and in all places.
- Many physical theories, such as Maxwell's laws of electrodynamics, and Einstein's theory of relativity, are deeply rooted in symmetry.
- >Top Internal symmetry:
- tells us the physical laws act the same way on distinct, but effectively indistinguishable, objects.
- Particle physics; treat particles and the fields that create them as interchangeable. (flavor symmetry)
- Sometimes exploiting even slightly imperfect symmetries helps us to compute sufficiently accurate results.
- Gauge bosons, Particles, and Symmetry:
- Each of the internal symmetries can be preserved only if it transforms both the gauge bosons and the particles with which they interact.
- Rotations wouldn't be symmetry transformations if the acted on some objects but not others. If you rotate the top wafer of an Oreo cookie, but not the rest of it, you would pull it apart. The Oreo cookie would look the same after a rotation only if you weer to rotate the entire thing simultaneously.
- The force that will interest us most is the weak force. the internal symmetry associated with the weak force treats the three weak gauge bosons as equivalent.
- It also treats particles pairs such as electron and neutrino, or up and down quarks, as equivalent.
- Symmetries are important to the theory of forces because the simplest workable theory of forces includes a symmetry associated with each force.
- Those symmetries eliminate unwanted particles. They also eliminate the false predictions that the simplest theory of forces would otherwise make about high-energy particles.
9. 対称性:
- Oreo Cookie:
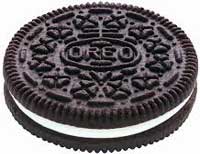
>Top10. The origin of elementary particle masses: Spontaneous symmetry breaking and the Higgs mechanism
- Symmetries are important, but slightly imperfect symmetries are what makes the world interesting. (Symmetry is broken)
- In physics, as in art, simplicity alone is not necessarily the highest goal. Life and the universe are rarely perfect, and almost all symmetries are broken.
- The goal is to make theories that are richer and sometimes even more beautiful without compromising their elegance.
- The concept of the Higgs mechanism, which relies on the phenomenon of spontaneous symmetry breaking.
- Scottish physicist Peter Higgs, lest the Standard Model particles acquire mass.
- Without the Higgs mechanism, all elementary particles would have to be massless.
- Spontaneously Broken Symmetry:
- Spontaneous symmetry braking is not only ubiquitous in physics, but is a prevalent feature of everyday life.
- occurs when all physical laws a symmetry but the actual physical system does not. Spontaneously broken symmetries are symmetrices that are preserved at high energyies but broken at low energies. The weak force symmetry is spontaneously broken.
- E.g.: a number of people are seated around a circular table with water glasses placed between them. Which glass should someone use?
- But one has to be chosen, and after that, left and right are no longer the same in that there is no longer a symmetry that interchanges the two.
- >Top Higgs Mechanism:
- Each type of field generates its own particular type of particle.
- An electron filed is the source of electrons. Similarly, a Higgs field is the source of Higgs particles.
- As with heavy quarks and leptons, Higgs particles are so heavy that they aren't found in ordinary matter.
- Higgs particles are too heavy to have been produced with the energies that experiments have explored so far.
- Higgs will be created within a few years, when the highter-energy LHC collider comes into operation.
- A non-zero Higgs field distributes weak charge throughout the universe. It is as if the nonzero weak-charge-carrying Higgs field paints weak charge throughout space.
- The vacuum itself carries weak charge when one of the two Higgs fields takes a nonzero value.
- Weak gauge boson interact with this weak charge of the vacuum, just as they do with all weak charge.
- The Higgs field plays a role very similar to that of the traffic cop in the story, restricting the weak force's influence to very sort distances.
- The weak charges in the vacuum have a density that corresponds roughly to charges that are separated by $10^{-15}$ cm (1 femto).
- With this weak charge density, the masses of the weak gauge bosons ($W^-, W^+, Z$) take their measured values of approximately 100 GeV (126 GeV as the Standard Model)
- Quarks and leptons interact with the Higgs field distributed throughout space and are therefore also hindered by the universe's weak charge.
- Photon is the only gauge boson involved in the electroweak force that is impervious to the weak charge of the vacuum.
- The chief distinguishing feature of the photon is that it travels unfettered through the weakly charged vacuum and therefore has no mass.
10. 素粒子質量の起源:
>Top11. Scaling and Grand Unification: Relating interactions at different lengths and energies.
- Grand Unified Theory (GUT), in which the three nongravitational forces merge into a single unified force at high energies.
- the unification energy is about 1000 trillion GeV, and the Planck scale energy, where gravity gets strong, is about a 1000 times greater than that.
- Zooming in and out:
- One advantage of an effective field theory is that even if you don't know what interactions take place over short distances, you can still study the quantities that matter at the scales that interest you.
- When you mix paint, you don't need to know its detailed molecular structure. But you probably want to know the properties that you readily perceive, like color and texture. However, if you did know your paint's chemical composition, the rules of physics would allow you to deduct some of those properties.
- Similarly, if you don' t know the short-distance (high energy) theory, you won't be able to derive measurable quantities. However, when you do know the short-distance details, quantum field theory tell you precisely how to relate the different effective theories that apply to different energies.
- 1974 Renomalization group; by Kenneth Wilson;
- When we curled up dimensions, we ignored all the details of what happened inside the extra dimensions and assumed that everything could be described in lower-dimensional terms.
- That way, you can neglect heavy particles that you'll never produce and short-distance interactions that will never occur. Instead, you can focus your calculations on particles and interactions that are relevant at the energy you can achieve.
- However, if you do know the more precise theory that applies at smaller distances, you can use that information to calculate quantities in the effective theory that interests you.
- >Top Virtual Particles:
- They pop in and out of existence, lasting only the barest moment.
- A particle moving very fast clearly carries a lot of energy. A virtual particle can have enormous speed but no energy. Virtual particle are a strange feature of quantum field theory that you have to include to make the right predictions.
- The uncertainty principle tells us that it would take infinitely long to measure energy (or mass) with infinite precision, and that the longer a particle lasts, the more accurate our measurement of its energy can be.
- Vacuum as a reservoir of energy - virtual particles are particles that emerge from the vacuum, temporarily borrowing some of its energy. They exist only fleetingly and then disappear back into the vacuum, taking with them the energy they borrowed.
- All particle-antiparticle pairs can in principle be produced, albeit only for very short visits, too short to be seen directly.
- A real physical photon can turn into a virtual electron and a virtual positron, which can then turn back into a photon. (>Fig.)
- Why interaction strength depends on distance:
- >Top Anarchic principle:
- follows from quantum mechanics, which tells us that all particle interactions that can happen will happen. In quantum field theory everything
- Physical particles can turn into virtual particles, which can interact with each other and then turn back into other physical particles. In such a process, the original physical particles might reemerge or they might turn into different physical particles.
- Even though the virtual particles wouldn't last long enough for us to observe them directly, they would nonetheless affect the way real observable particles interacted with one another.
- Not only direct interactions between physical particles, but also indirect interactions - those that involve virtual particles - play a role in communicating forces.
- Virtual correction to electron-positron scattering: (>Fig.)
- An electron and a positron annihilate into a photon, which in turn splits into a virtual electron-positron pair which then annihilate back into a photon, which in turn coverts to an electron and positron. The intermediate virtual electron and positron thereby affect the strength of the electromagnetic force.
- Grand Unification Theory (GUT):
- 1974 Georgi-Glashow:
- "We present a series of hypotheses and speculations leading inescapably to the conclusion ... that all elementary particle forces (strong, weak, and electromagnetic) are different manifestations of the same fundamental interaction involving a single coupling strength."
- GUT predicts that the proton decays. After a very long time, they would decay.
- a single high-energy force turns into the three known nongravitational forces at low energies. For the three forces to unify they must have the same strength at high energies.
- The net number of quarks in the universe would not remain the same, and a quark could change into a lepton, making the proton decay.
- the decay rate of the proton is very slow - the lifetime would far exceed the age of the universe.
- All familiar matter would ultimately be unstable.
11. スケーリングと大統一:
- Virtual Particle:
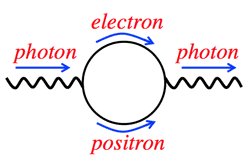
- Virtual correction to electron-positorn scattering:
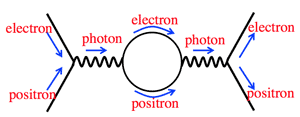
>Top 12. The Hierarchy Problem; the only effective trickle-down theory:
- the Standard Model predictions:
- The masses and charges associated with the electromagnetic, weak, and strong forces have been tested to incredbly high accuracy.
- Experiments at the colliders at CERN, SLAC (Stanford), Fermilab (Illinois) have all confirmed with exquiste precision the Standard Model predictions.
- the Higgs mechanism explains how the vacuum breaks electroweak symmetry and gives masses to the W and Z gauge bosons, as well as the quarks and the leptons.
- >Top The hierarchy problem in a GUT:
- the masses are not at all the same; even those particles that experience similar forces must have enormously different masses; not by a factor of ten, but of ten trillion.
- the new particle in GUT has to be extremely heavy.
- in a GUT; the weak force and the strong force should be interchangeable at high energy.
- the strongly charged particle that is partnered with the Higgs particle can interact simultaneously with a quark and a lepton and thereby enable the proton to deay.
- To avoid too rapid a decay, the strongly interacting particle must be extremely heavy; about one million billion GeV.
12. 階層問題:
- A contribution to the Higgs particle's mass:
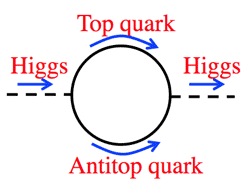
- The hierarchy problem:
Energy
Length
$10^{27}$GeV
Planck scale
$10^{18}$GeV
$10^{-33}$cm
$10^{15}$GeV
16 orders of magnitude
$10^{-30}$cm
:
:
$10^3$GeV
(TeV)
weak scale
$10^{-15}$cm
GeV
proton mass
$10^{-12}$cm
$10^{-3}$GeV
(MeV)
electron mass
$10^{-9}$cm
$10^{-6}$GeV
(keV)
>Top 13. Supersymmetry: A leap beyond the Standard Model
- "Super" words abound in physics; superconducting, supercooling, supersaturated, superfluid, Super conducting Supercollider (SSC)
- Supersymmetry was truly surprising.
- Supersymmetry as a symmetry of nature is still hypothetical.
- In a supersymmetric world every known particle is paired with another (superpartner), with which it is interchanged by a supersymmetry transformation.
- Fermions have half integer spin, while bosons have integer spin.
- such asymmetry defies logic. Symmetry transformation are supposed to leave system unchanged. But supersymmetry transformations interchange particles that are manifestly different; fermions and bosons.
- Superpartners, should they exist, must be more massive than their Standard Model partners. Because high-energy colliders might not yet have had enough energy to produce them. This would explain why we have not yet seen them.
- Once supersymmetry is broken, flavor-changing interactions can occur. These are processes that change quarks or leptons into quarks or leptons of another generation with the same charges.
- These are very strange processes; they change the identity of known particles, and they occur only rarely in nature. But most theories of broken supersymmetry predict that they should occur very often.
- Superhistory:
- 1971: Pierre Ramond; put forward the first supersymmetric theory; contained 2D supersymmetry and evolved into the fermionic string theory.
- 1973: Julius Weess, Bruno Zumino; developed 4D supersymmetric theory.
- Dmitri Volkov and Vladimir Ocelot in USSR independently derived another 4D supersymmetric theory.
- 1974: Sergio Ferrara and Bruno Zumino; formalism of superspace; but did not yet include gravity.
- 1976; Sergio Ferrara, Dan Freedman, and Peter van Nieuwenhuizen; solved by constructing supergravity
- Ferdinando Gliozzi, Joel Scherk, and David Olive; discovered a stable string theory. as an fermionic string theory.
13. 超対称性:
- Supersysmmetric partners:
Particle
Supeerpartner
lepton
slepton
electron
selectron
quark
squark
top
stop
gauge boson
gaugio
photon
photino
W bozon
wino
Z bozon
zino
gluon
gluino
graviton
gravitino
>Top 14. Allegro (Ma Non Troppo) Passage fro Strings:
- Incipient Unrest:
- Quantum mechanics and general relativity peacefully coexist over a wide range of distances.
- These two very different theories never adequately negotiated the extremely tiny distance as the Planck scale $10^{-33}$ cm.
- The gravitational force law tells us that on even tinier scales, the force of gravity is enormous; at the Planck scale length, gravity cannot be ignored.
- >Top At the Planck scale length, instead of a gradually undulating geometry there should be wild fluctuations and loops and handles of spacetime branching off.
- Given the conflict between them, there is no choice but to bring in an external arbiter as an alternative to both.
- The graviton is the only known massless particle whose spin is 2 - not 1 as for other gauge bosons, or $\frac{1}{2}$ as for quarks and leptons.
- Current theories of cosmology conjecture that the universe began as a tiny ball; the bang of the Big Bang.
- Black hole's horizon and at the singularity, the place in the center of the black hole.
- String training:
- Strings - vibrating, 1D loops or segments of energy.
- We now believe that string theory can contain different, independent types of string, each of which can oscillate in many possible ways
- strings are 1D objects.
- two type of string; open strings, having two endpoints, and closed strings, are loops with no ends.
- For both open and closed strings, the resonant modes are those that oscillate an integer number of times along the string's length; any other waves - those that don't complete an integer number of oscillations won't occur.
- the string oscillates determines all of a particle's properties, such as its mass, spin, and charge.
- Known particles, which are relatively light, arise from strings with the fewest oscillations.
- a mode with no oscillations could be a familiar light particle, such as ordinary quark or lepton.
- >Top Superstring Theory:
- it contained spin $-\frac{1}{2}$ particles, giving it the potential to describe the Standard Model fermions such as electron and quarks.
- it did not contain the tachyon that had plagued the original string theory.
- the spin-2 particle behaved just as a graviton should.
- Superstring Revolution:
- Strings could conceivably move around in 3, r, or more dimensions. Calculations indicated that the correct number (including time) was 10.
- 1969: Steven Adler, et al, showed that even when a classical theory preserves a symmetry, quantum mechanical processes involving virtual particles sometimes violate that symmetry; as called anomalies.
- Virtual quarks and leptons would lead to anomalous quantum contributions that would break the symmetries of the Standard Model.
- However, the sum of the quantum contributions from the quarks and the leptons adds up to zero.
- 1980: Schwarz and Michael Green; worked out the subsequences of the superstring; known as anomalies.
- String theory was largely ignored until 1984, the year that Green and Schwarz demonstrated a starling feature of the superstring which convinced many other physicists that they were on a promising track.
- 1985:
- Princeton collaboration of David Gross, et al. derived a theory of heterotic string;
- In string theory, a vibrational mode can move either clockwise or counterclockwise along the string. "Heterotic" was used because waves moving to the left were treated differently from those waves moving to the right.
- 1985, Philip Candelas, et al.; complicated way of curl up the extra dimensions, namely a competitiveness known as Calabi-Yan manifolds.
- >Top 1980s: Theory of Everything (ToE);
- String theory was more ambitious even than Grand Unified Theories; with string theory, physicists hoped to unify alll forces (including gravity) at an energy higher even tthan the energy associate with GUTs.
- Aftermath of the Revolution:
- Even in the absence of particles, the universe can carry energy known as vacuum energy.
- Positive vacuume energy accelerated the expansion of the universe, while negaive energy makes it collapse.
- Einstein first proposed such an energy in 1917 in order to find a static solution to his equations of general relativity.
- Astronomers have recently measured the vacuum energy in our cosmos (dark energy or the cosmological constant) and found a small positive value.
- The supernova measurements tells us that the expansion of the universe is accelerating.
- String theory has yet to explain why the universe's vacuum energy is as small as we know it must be.
- Nonetheless, string theory is a remarkable theory. It has already led to important insights into gravity, dimensions, and quantum field theory and it's the best candidate we know of for a consistent theory of quantum gravity.
- Model builders are still waiting for the experimental clues that tell us which of the myriad possibilities correctly describe physics beyond the Standard Model; energy higher than a TeV.
14. 急速な紐の通路:
- String oscillation models:
Open string and Closed string
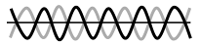
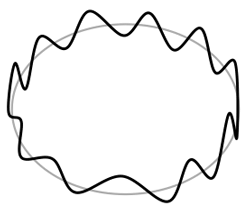
- String Theory:
- Superstring theory:
- Spin-2 particle=graviton?
>Top 15. Supporting Passages; Brane development
- Branes:
- String theory is no longer just the theory of strings extending in one spatial direcrion, but also the theory of ranes that can extend in 2, 3, or more dimensions.
- We now knw that branes, which can extend in any number of dimensions up to the number that supersting theory contains, are just as much a part of superstirng theory as are strings themslves.
- >Top M-theory: began in 1980s and developed in 1990s.
- an 11-dimensional theory that embraces both superstring theory and 11D supergravity.
- M stands for membrane, magic, mystery, Missing theory.
- Duality:
- is one of the most exicitng conecpts of the last ten years in particle physics and string theory.
- in 1977, Claus Montonen, et al. showed that a particular theory remained exactly the same if the particles and strings of the theory were interchanged.
- >Top Perturbation theory;
- lest you creep up on the answer to a question in the weaky interacting theory by starting from the thoery with no interactions and calculatin small improvements in incremental stage.; to refine a claculation in successive steps until you reach any desired level of precision.
- More on duality:
- 10D supergravity does not contain strings, whereas 11D supergravity does not. Even though 11D supergravity does not contain strings, it does contain 2-branes. But ulike strings, which have only one spatial dimension, 2-branes have two.
- Now, suppose that one of the 11D is rolled up into an extremely tiny circle; 2-brane that encircles the rolld-up circular dimension looks just like a string.
- This means 11D supergravity theory with a rolled-up dimension appears to contain strings.
- A rolled-up dimension is invisible at long distances or low energies. (>Fig.)
15. 脇道の通路:
- Rolled-up brane:
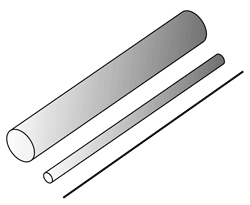
>Top 16. Bustling Passages; Braneworlds
- Particles, Strings, and Branes:
- A string that begins and ends on a single brane can give rise to a gauge boson.
- A string with each end on a different brane gives rise to a new type of gauge boson. When the brans are separated, the gauge boson has nonzero mass.
- the mass of the particles arising from the vibrational modes of this string grows with the distance between the branes. This mass is like the energy that gets stored when you stretch a spring.
- Now imagine that many branes are superimposed. there would then be many new types of open string because the two string ends can be confined to any of the branes.
- Open strings that extend between different branes, or the strings that begin or end on any single one of the branes, imply new particles, composed of the vibrational modes of these strings. (>Fig.)
- Particles on separated branes don't interact with each other directly. Interactions are local; they can take place only among particles in the same place. particles on separated branes would be too far apart to interact with each other directly.
- E.g.: a huge tennis stadium with separate matches going on throughout. Each ball would stay on it own isolated court.
- Gravity:
- Gravity, unlike all other forces, is never confined to a brane.; graviton is a mode of a closed string.
- Closed strings have no ends, and therefore there are no ends to pin down on a brane.
- Graviton, unlike gauge bosons or fermions, must travel though the entire higher dimensional spacetime. there is no way to confine gravity to lower dimensions.
- Model Braneworlds:
- Brane scenarios introduced many new possibilities for the global nature of spacetime.
- As of now, string theory doesn't tell us whether brans exist and, if they do, how many there are. We know only that branes are an essential theoretical piece of string theory.
- If Standard Model particles are confined to a brane, then we are as well, since we and the cosmos that surrounds us are composed of these particles.
- There might therefore be entirely new and unfamiliar particles that experience different forces and interactions from the ones we know.
- The particles and forces we observe might be only a small part of a much larger universe.
- >Top Hořava-Witten Theory:
- " branes with 9 spatial dimensions are separated along the 11th spacetime dimension (10th spatial dimension). The bulk includes all spatial dimensions; those 9 that extend in the spatial directions along the two branes, and the additional one that extends between them. (>Fig.)
- 11th dimension is not rolled up, but is instead bounded between two branes.
- If the HW braneworld is to correspond to reality, size of tis dimension must be unseen. HW assumed that six dimensions were curled up into a tiny Calabi-Yau shape.
- it can accommodate not only the Standard Model particle and forces, but also a full GUT. Because gravity originals in higher dimensions, it's possible for gravity and other forces to have the same strength at high energy in this model.
- Why braneworlds can matter for real-world physics.
- More than a single brane: can contain forces and particles that interact with each other only weakly because of the distance between the two branes.
- Any braneworld introduces new length scales into physics; like the size of the additional dimensions, might be relevant to unification or the hierarchy problem.
- Branes and the bulk can carry energy.; it doesn't depend on the particles that are present.
16. 賑やかな通路:
- Strings on the brane:
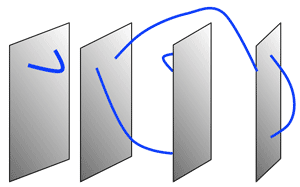
- Hořava-Witten Theory:
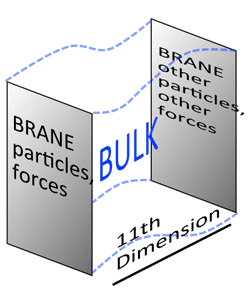
>Top 17. Sparsely populated passages; multiverses and sequestering:
- Sequestering; extra dimensions could prove to be important for particle physics.
- sequestering might also be the reason that the anarchic principle, which says that everything should interact, doesn't always hold true.
- If particles are separated in extra dimensions, they are less likely to interact with one another.
- Extra dimensions introduce a way to separate particles.
- Whereas 4D theories face serious problems because supersymmetry-breaking models generally introduce unwanted interactions, sequestered supersymmetry-breaking models appear to be far more promising.
- Sequestering might also explain why particles have different masses from one another, and why proton decay does not occur in extra-dimensional models.
- "Natural" : for physicists, it is only natural to expect the expected.
- Symmetries essentially provide an extra rule about which interactions can conceivably happen.
- Suppose that you prepare six table setting; permit a symmetry transformation that interchanges every pair of setting; all six people have the same number of forks, knives, spoons, and chopsticks.
- Particles on different branes;
- don't interact strongly because interactions are always local; only if there are interacting particles that can travel from one brane to the other.
- This suppression of interactions between particles sequestered in different places would be similar to the suppression of International information in a country. (xenophobia)
17. 過疎の通路:
- Superesymmetry breaking:
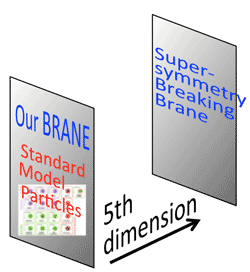
>Top 18. Leaky passages; Fingerprints of extra dimensions
- Kaluza-Klein particles:
- New particles that originatd in extra dimensions, but appear to us as extra particles in our 4D spacetime, are Kaluza-Klein particles.
- The relationship between mass and momentum imposed by special relativity tells us that extra-dimensional momentum would be seen in 4D world as mass.
- Determining Kaluza-Klein Masses:
- The extra-dimensional momenta that the KK particles carry would appear to us in our apparently 4D word as a distinctive pattern of KK particle masses.
- Quantum mechanics associates all particles with waves, and only those waves that can oscillate an integer number of times over the extra-dimensional circle are allowed.
- The lightest KK particle is therefore the one associate with this constant probability value in the extra dimension.
- Heavier KK particles, which carry nonzero extra-dimensional momenta, will be the first real evidence of extra dimensions.
- The wavelength is determined by the size of thee extra dimension's circumference.
- the lighter KK particle that "remembers" its extra-dimensional origin.
- Kaluza-Klein particles correspond to the waves that oscillate an integer number of times around the curled-up dimension.
- Waves with more oscillations correspond to heavier particles.
- KK particles and their masses could tell us quite a lot about extra-dimensional properties. (extra dimensions' sizes and shapes)
- KK particles resemble the multiple generations of an immigrant family; Younger generation born in US fully assimilate American culture, and don't betray their foreign roots.
- Experimental Constraints:
- Until recently, most string theorists assumed that extra dimensions are no bigger than the minuscule Planck scale length ($10^{-33}$ cm)
- Perhaps extra dimensions are bigger and KK particles are lighter. Why not ask instead what experimental tests tell us about an extra dimension's size?
- So far there has not been any sign of such charged particles at colliders. (about TeV scale about $10^{-17}$ cm)
- Current experimental constraints tell us that extra dimensions cannot be any larger than $10^{-17}$ cm. This is extremely small, about 10 times smaller than the weak scale length.
- But it is huge compared with the Planck scale length, 16 orders of magnitude small.
- This means that extra dimensions could be much bigger than the Planck scale length and sill have evaded detection.
18. 漏れる通路:
- Kaluza-Klein theory:
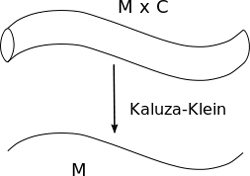
- Kaluza-Klein praticles:
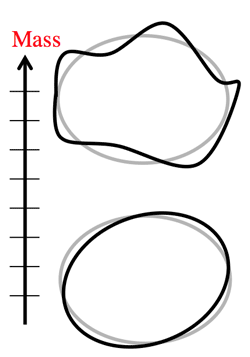
>Top 19. Voluminous Passages; Large extra dimensions
- 1998: Arkani-Hamed, Dimopoulos, and Dvali (ADD):
- How very large dimensions might explain the weakness of gravity.
- ADD wrote: extra dimensions could be as big as a millimeter (or even 10 times smaller).
- Branes can trap quarks, leptons, and gauge bosons so that only gravity experiences the full higher dimensionality of space.
- Extra dimensions can be so large that they can explain why gravity is so much weaker than the electromagnetic, weak, and strong forces.
- In the ADD scenario, which assumes that everything other than gravity is confined to a brane, everything that doesn't involve gravity wold look exactly the same as it would without the extra dimensions, even if the extra dimensions were extremely large.
- Your eye detects photons, and photons in the AD model are trapped on a brane. Therefore all objects you see would look as if there were only three spatial dimension.
- In the ADD model, the only particle that must have KK partners is the graviton, which must travel in the higher dimensional bulk.
- Until recently, the main question that why the weak scale mass is so small, despite the large (Planck-scale-mass-size) virtual contributions to the Higgs particle's mass that tend to make it larger.
- They proposed that the fundamental mass scale that determines gravity's strength is not the Planck scale mass, but a much small mass scale, close to a TeV.
- After all, the reason that the Plank scale mass is so big is that gravity is weak - gravity's strength is inversely proportional to this scale. A much smaller fundamental mass scale for gravity would make gravitational interactions far too strong.
- Back to the hierarchy problem:
- Suppose that gravity in a higher dimensional theory doe not depend on the enormous Plank scale mass of $10^{19}$ GeV, but instead on a much smaller energy, about a TeV, 16 orders of magnitude smaller.
- They chose a TeV to eliminate the hierarchy problems; if a TeV or some nearby energy were the energy at which gravity became strong, there would be no hierarchy of masses in particle physics. Everything would be characterized by the TeV scale. So maintaining a reasonably light Higgs particle with mass of about a TeV would not be a problem in their model.
- Collider searches for large extra dimensions:
- If the large-dimension proposal applies to the real world, the graviton KK partners would be light enough to be produced at accelerators, no matter how many extra dimensions there were.
- Current colliders create more than enough energy to make such low-mass particles.
- If there were two extra dimensions, about 100 billion trillion KK modes would be light enough to be produced at a collider operation at an energy of about a TeV.
- The rate of producing at least one of these particles would be fairly high, even in the rate of producing any single one of them were extremely low.
- It would be as if someone hinted something to you in such a subtle manner that you didn't take it to heart the first time you heard it. But afterwards, 50 people repeated the same thing. Even though you wouldn't take much notice the first time you heard the messaged, by the 50th time the message would register.
- The large Hadron Collider, which will study TeV-scale energies, could produce KK particles at a measurable rate if the ADD idea is correct.
- KK particle production in the ADD model (>Fig.)
- Protons collide, and a quark ad an antiquark annihilate into a virtual gluon. The virtual gluon turns into an undetected KK particle and an observable jet. The gray lines are sprays of additional particles that protons always emit when they collide.
19. たっぷりした通路:
- KK particle production in the ADD model:
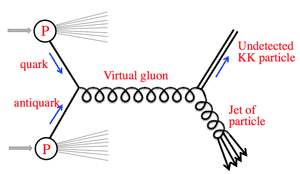
>Top 20. Warped Passage: A solution to the hierarchy problem
- The geometry that we'll consider here contains 2 branes that bound a 5D of space (>Fig.)
- The universe has 5 spacetime dimensions, but the Standard Model resides on a brane (the Weakbrane) that has four.
- The total number of spacetime dimensions.
- There are 2 btanes with a 5th dimension that etends between them.
- The particles and the distribution of energy are different, and the theory is not supersymmtric.
- We assue that all of the Standard Model particles, along with a Higgs particle responsible for breaking electroweak symmetry, are confined to one of the two branes.
- W'll assume that gravity is the only forrce that exists throughout the 5th dimension.
- Each of the branes would look like a conventional 4D universe. Gauge bosons and particles confined to the branes would communicate forces and interact as if the 5D didn't exist.
- Gravity is different since it is not restricted to a brane, ubt instead exists in the full 5D bulk. But this does not necessarily mena that is is felt equally everywhere.
- Energy o nbt ebranes and int 5D bulk curves spacetime, and this makes an enourmous difference to the gravitational field.
- The 5D spacetime is nonetheless curved.
- The warp factor is a function that changes the overall scale for position, time, mass, and energy at each point in the 5D.
- A curvd space iwth flat slices is pictured in Fig. It is a filled-in funnel.
- Ths curvature would be reflectd in an overall rescaling of the measuring rode of space and the clock spd for time, which would be different at each point in 5D.
- The graviton si shte particle that communicates the gravitational force, and its probaility function tells us the likelihood of finding the graviton at nay fixed position in space.
- The graviton's probability unction alls of exponentially as it move away from the Gravitybrane and twards the Weakbrane.
- The distance between branes in this warped geometry need only be a little larger than the Planck scale length.
20. ワープした通路:
- Warped 5D geometry with 2 branes:
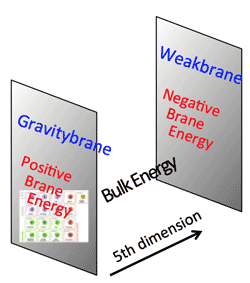
- A filled-in funnel:
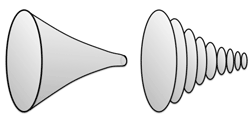
>Top 21. Warped Annotated "Alice":
- The tittle borrows from Martin Gardner's delightful Annotated Alice, in which he explains the wordplay, math riddles, and references in Lewis Carroll's Alice in Wonderland and Through the Looking Glass.
- The brane itself is large and flat and has only three spatial dimensions. Only gravity makes contact with the additional dimension.
- Remember that 5D space had 4 spatial dimensions and one of time, whereas the brane has 3 spatial dimensions. I'll still call time the 4th dimension, and I'll call the additional dimension the 5th.
- The Fat Cat, unlike Branesville residents, is not confined to the brane.
- Everything is bigger and lighter near the Weakbrane. Athena's shadow over Branesville grew as she got close to the Weakbrane and further away from the Gravitybrane.
- The 5th dimension does not have to be very big in order to solve the hierarchy problem.
- Gravity is feeble on the Weakbrane, where the graviton's probability function is so small.
- On the Gravitybrane, gravity is no weaker than the other forces.
- The petulant graviton is complaining that on the Weakbrane, gravity is much weaker than the electromagnetic, weak, and strong forces. Gravity would be much stronger closer to the Gravitybrane.
- Things are bigger and time is slower on the Weakbrane. the rabbit's laxness is accounted for by rescaling time.
21. ワープした注釈付きのアリス:
>Top 22. Profound Passage; An infinite extra dimension:
- The localized graviton:
- the localized graviton has unrestricted access to an infinite 5D. But it is nonetheless highly concentrated in the vicinity of a brane, and has very low probability of being found far away.
- Because the graviton only rarely ravels outside a limited region, the extra dimension can be infinite without giving rise to any dangerous effects that would rule the theory out.
- Kaluza-Klein Partners of the Graviton:
- Graviton travels almost exclusively along the brane and has only a tiny probability of leaking out into 5D.
- From the graviton's perspective, space looks as if the 5D is on $10^{-33}$ cm in size, rather than of infinite extent.
- In localized gravity, the massless KK particle is the localized graviton. It is concentrated close to the Gravitybrane.
- All other KK particles are concentrated far from the Gravitybrane; the shape of their probability function and the locations where they peak depend on their mass.
22. 深淵な通路:
- Graviton's probability function in infinite warped spacetime with a single brane:
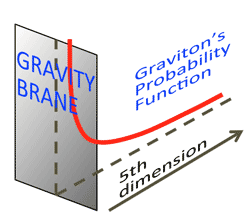
>Top 23. A Reflective and Expansive Passage:
- Reflections:
- After our theory was accepted and no longer thought incorrect, some physicists actually went overboard in a different direction, claiming our theory was nothing new.
- Localized gravity turned out to have strong overlaps with the most important string develpments of the time; both our work and the research of string theorists involved a similarly warped geometry.
- Locally Localized Gravity:
- how many dimensions of space are there?
- You now know that extra dimensions can be hidden either because there are curled up and small, or because spacetime is warped and gravity so concentrated in a small region that even an infinite dimension is invisible. Either way, whether dimensions are compact or localized, spacetime would appear to be 4D everwhere, no matter where you are.
- Gravity acts as it does in 4D if you're near the brane.
- Although the graviton's probability function is largest on the Gravitybrane, objects everywhere can interact with one another by exchanging a graviton, and therefore all objects would experience 4D gravity, independently of location.
- Gravity everywhere looks 4D because the graviton's probability function is never actually zero - it continues on for ever.
23. 収縮・膨張する通路:
>Top 24. Extra Dimensions; are you in or out?
- Warped geometry and Duality:
- how can a 4D and a 5D (or 10D) theory have the same physical implications?
- The answer is that an object moving through 5D would appear in the dual 4D theory as an object that grows or shrinks. this is just like
- Athena's shadow on the Gravitybrane, which grew as she move away from the Gravitybrane across the 5D. Furthermore, object moving past each other along the 5D correspond to objects that grow and shrink and overlap in 4D.
- T-duality:
- it exchanges a space with a tiny rolled-up dimension for another space with a huge rolled-up dimension. Odd as it may seem, in string theory, extremely small and extremely large rolled-up dimensions yield the same physical consequences.
- Mirror Symmetry:
- T-duality applies when a dimension is rolled up into a circle. But an even weirder symmetry than T-duality is mirror symmetry, which sometimes applies in string theory when 6D are rolled up into a Calabi-Yau maniforld.
- Mirror symmetry says that 6D can be curled up into two very different Calabi-Yau manifolds, yet the resulting 4D ling-distance theory can be the same.
- Matrix Theory:
- is a tool for studying string theory, provides still more mysterious clues about dimensions.
- Superficially, it looks like a quantum mechanical theory that describes the behavior and interactions of Do-branes (pointlike branes) moving through 10D.
- Furthermore, the theory of Do-branes mimics supergravity in 11D, not 10D.
- What to think?:
- No one knows what the best description would be when a dimension is neither very big nor very small. Perhaps our notion of spacetime breaks down altogether once we try to describe something so small.
- We know from quantum mechanics that it takes a lot of energy to investigate small length scales. But once you put too much energy into a region as small as the Planck scale length, you get a black hole; then not way to know what's happening inside.
24. 余剰次元:
>Top 25. Conclusion:
- We don't yet know how to harness the force of gravity or teleport objects across space.
- We don't know how to connect universes in which you could loop through time to the one in which we live; no one can create a time machine.
- Our goal is to learn how its pieces fit together and how they've evolved into their current state. What at the connections that we haven't yet figured out?
- Even if we have yet to understand the ultimate origin of matter at the deepest level.
- Even if we don't know the most basic elements of spacetime, we do understand its properties for distances far away from the Planck scale length.
- Physicists are now developing detailed theories of black holes in extra-dimensional worlds.
- Cosmological observations might also ultimated tell us more about the structure o of spacetime.
- If we live in a higher-dimensional universe, it must have been very different earlier on.
- We might learn about dark matter hidden on other branes, or cosmological energy stored by hidden higher dimensional objects.
- the Large Hadron Collider (LHC) at CERN will turn on and probe physical regions no one has ever observed before.
- LHC will have enough energy to produce the new types of particle; superpartners or other particles that 4D models predict.; also be Kaluza-Klein particles.
- The universe is about to be pried open; Astrophysical observations; Discoveries at the LHC.
25. 結論:
Comment
- a
>Top 8. Experimental Interlude; Verifying the Standard Model:
|
8. 実験的な幕間:
|
>Top 9. Symmetry:
|
9. 対称性:
|
>Top10. The origin of elementary particle masses: Spontaneous symmetry breaking and the Higgs mechanism
|
10. 素粒子質量の起源: |
>Top11. Scaling and Grand Unification: Relating interactions at different lengths and energies.
|
11. スケーリングと大統一:
|
>Top 12. The Hierarchy Problem; the only effective trickle-down theory:
|
12. 階層問題:
|
>Top 13. Supersymmetry: A leap beyond the Standard Model
|
13. 超対称性:
|
>Top 14. Allegro (Ma Non Troppo) Passage fro Strings:
|
14. 急速な紐の通路:
|
>Top 15. Supporting Passages; Brane development
|
15. 脇道の通路:
|
>Top 16. Bustling Passages; Braneworlds
|
16. 賑やかな通路:
|
>Top 17. Sparsely populated passages; multiverses and sequestering:
|
17. 過疎の通路:
|
>Top 18. Leaky passages; Fingerprints of extra dimensions
|
18. 漏れる通路:
|
>Top 19. Voluminous Passages; Large extra dimensions
|
19. たっぷりした通路:
|
>Top 20. Warped Passage: A solution to the hierarchy problem
|
20. ワープした通路:
|
>Top 21. Warped Annotated "Alice":
|
21. ワープした注釈付きのアリス: |
>Top 22. Profound Passage; An infinite extra dimension:
|
22. 深淵な通路:
|
>Top 23. A Reflective and Expansive Passage:
|
23. 収縮・膨張する通路: |
>Top 24. Extra Dimensions; are you in or out?
|
24. 余剰次元: |
>Top 25. Conclusion:
|
25. 結論: |
Comment |
|