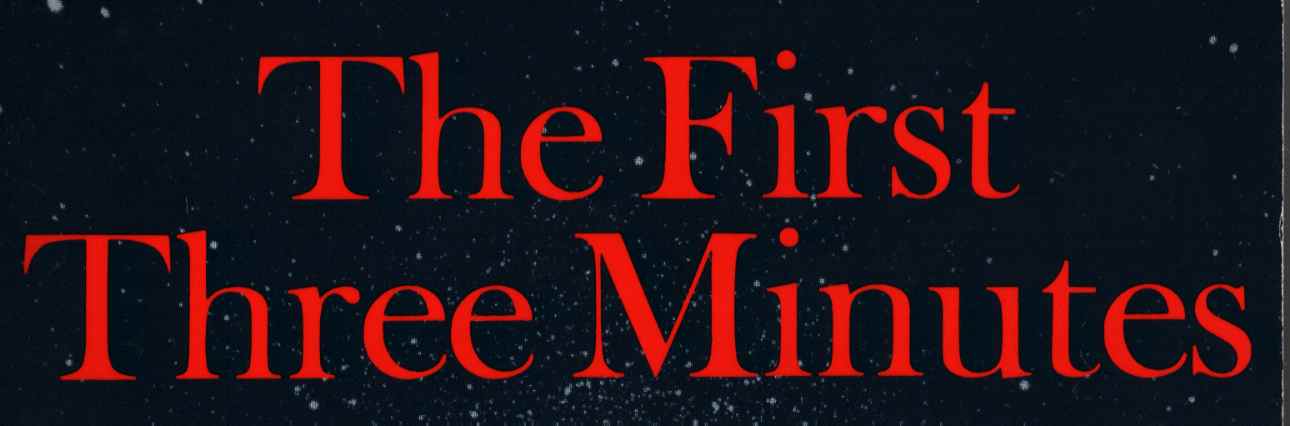
The First Three Minutes
A Modern View of the Origin of
the Universe
Cat: SCI
Pub: 1993
#: 9301b
Steven Weinberg
93131u/18221r
Title
The First Three Minutes
宇宙創生最初の3分間
Sub Title
A Modern View of the Origin of the Universe
宇宙創世に関する最新論
Author
Steven Weinberg
スティーブン・ワインバーグ
Published
1993
1993
Index
Tag
; 1 billion K after 3min; Black body radiation; Cepheid variable; Early universe; Isotropic & homogenoius; Hubble constant; Light neutrino antineutrino; Matter dominated; Radiation background; Radiation dominated; Violent explosion; ;
Why
this
book?
- The author is a winner of the 1979 Nobel Prize for Physics.
- He prefaces: "What could be more interesting than the
problem of Genesis? ... In just the last decade a detailed theory
of the course of events in the early universe has become widely
accepted as a standard model....it is exhilarating that we are now
able to speak of such things with any confidence at all..."
- 著者は1979年ノーベル物理学賞受賞者。
- 巻頭言「宇宙創世の問題より興味深い問題があるだろうか。過去10年に初期宇宙の出来事についての詳細理論広く受け入れられるようになった。今や、この問題について確信をもって話ができることは幸いである。」
Summary
要約
>Top 1. The Origin of the Universe:
- In 1950s, the study of the early universe was not regarded as a respectable
scientist would devote his time. There has not existed an adequate
observational and theoretical foundation.
- Now the standard model is widely accepted: 'big bang' theory.
>Top <Summary of the history of the early universe>:
- In the beginning there was an explosion.
(Note: The Old Testament says ".......")
This occurred simultaneously everywhere, filling all space from
the beginning, with every particle of matter rushing apart from
every other particle.
(all space = a finite universe which curves back on itself like
the surface of a sphere.)
- 1/100 of a second:
the temperature of the universe was about a hundred thousand million
($10^{11}$) Kelvin.
the elementary particles (like electron, positron, neutrino, and
photon) apart in this explosion.
- Density of the cosmic soup:
4 billion times that of water; a small contamination of protons
and neutrons. The proportions were roughly one proton and one neutron
for every billion electrons or positrons or neutrinos or photons.
- >Top The explosion continued the temperature dropped, reaching
- 30 billion K. after 1/10 second
- 10 billion K. after a second
- 3 billion K. after 14 seconds
- 1 billion K. after 3 minutes;
cool enough for protons and neutrons to form into complex nuclei
(deuterium, then helium)
- At the the end of the first 3 minutes:
the contents of the universe were mostly light, neutrinos, and antineutrinos,
with small amount of nuclear material of about 73% hydrogen and
27% helium and small number of electrons left over from electron-positron
annihilation.
- After a few hundred thousand years:
electrons to join with nuclei to form atoms of hydrogen and helium.
The resulting gas begins to form clumps, ultimately condense to
form galaxies and stars under the influence of gravitation.
1.宇宙の起源:
- 1950年代には、初期宇宙の研究に時間を費やすことは尊敬できる科学者とは見なされなかった。それはそれにふさわしい観測や理論の基礎がなかったからである。
- 今やビッグバン理論は標準モデルとして広く受け入れられている。
<初期宇宙の歴史のまとめ>:
- 初めに爆発があった。(注:旧約聖書には...)
この爆発は至る所で同時に発生し、すべての素粒子が互いに分離して全宇宙を満たした。(全宇宙とは、球の表面 の如くそれ自身が閉じた有限の宇宙)
- 1/100秒後:
宇宙の温度は$10^{11}$度K。
電子・陽電子・ニュートリノ・光子などの素粒子はこの爆発で分離。 宇宙スープの濃度:
水の40億倍で、若干の陽子と中性子を含む。比率は陽子、中性子各1個に対し、電子・陽電子・ニュートリノ・光子は各約10億個。 爆発の経過と共に温度が低下
- 1/10秒後: 300億度
- 1秒後: 100億度
- 14 秒後: 30億度
- 3分後: 10億度
この温度になると陽子、中性子からより複雑な原子核(重水素、ヘリウム)が生成
- 最初の3分間の終わり:
宇宙はほとんどは光・ニュートリノ・反ニュートリノからなり、若干の核種 (水素73%、ヘリウム27%)および電子−陽電子の対消滅の残った少量
の電子から成る。
- 数億年後:
電子は原子核と合体し水素やヘリウムを生成。
それらのガスはガスの塊となり、ついには重力の影響で銀河や恒星を生成。
Future of the universe:
- It may go on expanding forever, getting colder, emptier, and
deader.
- Alternatively, it may recontract, breaking up the the galaxies
and stars and atoms and atomic nuclei back into their constituents.
宇宙の将来:
- 永遠に膨張し、更に冷却し、空虚になり、そして死んでいく。
- あるいは、再び収縮に転じ、銀河、恒星、原子、原子核は素粒子にまで崩壊していく。
>Top 2. The expansion of the universe:
- <Proper motion>
The stars seem motionless. but they do move, at speeds of a few hundred
km/sec. Distant stars change so slowly that their proper motion cannot
be detected with even patient observation.
- This impression of changelessness is illusory. The universe is in
a state of violent explosion; galaxies are rushing apart almost at
the speed of light.
- Further we can extrapolate this explosion backward in time and conclude
the all the galaxies must have been much closer in the past - so close
that neither galaxies nor stars nor even atoms or atomic nuclei could
have had a separate existence; the era called "the early universe."
2.宇宙の膨張:
- <固有運動>
星は動かないように見えるが、実は秒速数Kmの速さで動いている。遠くの星の変化はゆっくりなので忍耐強く観察しても固有運動を検知することはできない。
- この恒星の不動という印象はまったくの幻想である。宇宙は実は激しい爆発の状態にある。銀河はほとんど光のスピードで離れている。
- この爆発を時間的に逆転させて推定すればすべての銀河は過去にはもっと接近していた。そしてついにはどのの銀河も恒星も、原子や原子核でさえ離れて存在することはできなかった。その時期のことを"初期宇宙"と呼ぶ。
>Top <Steps to know the expansion of the universe>
- <Doppler effect>
by Johann Christian Doppler in 1842
The light from moving away stars from the earth shifts toward longer
(redder) wavelength. Moving toward us shifts toward shorter (bluer).
- <Frauenhofer lines>
by Joseph Frauenhofer in 1814
Dark lines are produced by the selective absorption of light of
certain definite wavelengths.
- <Nebula>
by Charles Messier in 1781; published catalog of Nebulae and Star
clusters (Andromeda Nebula is M31, Crab Nebula is M1....)
- <Supernova>
Explosions of one star approaches the luminosity of a whole galaxy.
- >Top <Cepheid variables>
by Edwin Hubble in 1923;
a tight relation between the observed periods of variation of the
Cepheids and their absolute luminosities. And he concluded that
Andromeda Nebula is 900,000 light years (10 times farther) than
the most distant known objects in our galaxy.
(actually 2.54M ly) - <Extragalactic nebulae>
by Vesto Melvin Slipher in 1910-20;
Andromeda Nebula is moving toward the earth at 300 km/sec. But more
and more of the larger spectral shifts toward the red end were discovered.
- <Exploding universe>
by Hubble in 1929; observed a proportionality between the distances
of galaxies and their speeds of recession.
- >Top <Cosmological Principle>
The Universe seems remarkable isotropic and homogeneous; it looks
the same in all directions.
- <Hubble constant>
Hubble by 1931; verified the proportionality between velocity and
distance for galaxies and concluded that velocities increase by
170 km/sec for every million light years distance; thus, a velocity
of 20,000 km/sec means a distance of 120 million light years.
- >Top <Hubble constant - revised>
by Allan Sandage of Palomar and Mt. Wilson; about 15
km/sec per million light years. (Now: 70.0 km/s/Mpc)
The galaxies have not been moving at constant velocities,
but have been slowing down by mutual gravitation. Therefore, we
can refer the age of universe must be less than 20 billion years.
- <Age of our galaxy>:
Our galaxy is about 10-15 billion years old; calculated by
the rate of radioactivity (especially relative abundance of U-235
and U-238), stellar evolution and the red shift of distant galaxies.
- <Cosmological constant>
In 1917 Albert Einstein mutilate his equations by introducing
so-called cosmological constant to keep the elegance of the original
theory (Universe should be homogeneous, isotropic and static.
= Einstein's static universe)
This constant could serve to balance the attractive force of gravitation
at large distances.
- <Friedmann model>
in 1922 Alexandre Friedmann provided; If the average
density of the matter of the universe is less than a certain critical
value, then the universe will expand forever. Or if it is greater
than this critical value, then the gravitational fields are strong
enough to stop the expansion of the universe, so that it will eventually
implode back to indefinitely large density.
The critical density is proportional to the square of the Hubble
constant.
The critical density equals $5 \times 10^{-30}$ grams per cubic cm, or three
hydrogen atoms per 1000 litters of space.
- <Hubble diagram>
Expansion or Contraction of the Universe:
One way to tell whether or not the galactic velocities exceed escape
velocity it to measure the rate of slowing down. If this deceleration
is less (or greater) than a certain amount, then escape velocity
is (or is not) exceeded.
Luminosity-Distance (billion light years) is often called Hubble
diagram.
<宇宙膨張を知るための基礎>
- ドップラー効果:
Johann C. Doppler (1842)による。地球から遠ざかる星からの光の波長は長くなり (赤方偏移)近づく場合は波長が短くなる (青方偏移)
- フラウンホーファー線:
Joseph Frauenhofer (1814)による。一定の波長の光が選択的に吸収されて生じる暗線
- 星雲:
Charles Messier (1781)によってカタログとして発表された星雲および星団 (アンドロメダ星雲はM31、かに星雲はM1等)
- 超新星:
一つの星の明るさが銀河全体の明るさに匹敵するような大爆発。
- セファイド変光星:
Edwin Hubble (1923)により発見された脈動変光星 (最初に発見されたケフェウス座δ星に由来)。変光周期と真の明るさの間に周期‐光度関係と呼ばれる強い相関関係がある。これによってアンドロメダ星雲までの距離は90万光年として、銀河系のどの物体より10倍以上も遠方にあると結論した。(実際には254万光年)
- 銀河系外星雲:
Vesto Melvin Slipher (1910-20)による。アンドロメダ星雲は地球に向かって秒速300/kmで近づいている。さらにずっと大きな赤方偏移を示す星雲が発見された。
- 膨張宇宙:
Hubble (1929)は、銀河とその後退速度との間に比例関係を発見。
- 宇宙原理:
宇宙は大局的には等方的で一様であり. 中心はなく, どの点も対等となる。
- ハッブル定数:
Hubble (1931)は、銀河の距離と後退速度の間の比例関係を検証し、1百万光年毎に秒速170kmであると結論した。これは秒速2万kmは120百万光年に相当する。
- ハッブル定数 (修正):
Allan Sandage (Palomar and Mt. Wilson)は、1百万光年毎に秒速15kmと修正。銀河は必ずしも一定の速度で動いておらず、相互の重力によって速度が遅くなっている。その結果
宇宙の年齢は200億年以下と推定。
(2017現在は、70.0 km/s/Mpc)
- 銀河の年齢:
我々の銀河の年齢は、放射性元素の崩壊比率 (特に、U-235/U-238比)、恒星の進化および遠方銀河の赤方偏移から、100-150億年と計算。 - 宇宙定数:
Albert Einstein (1917)は、一般相対性理論の重力方程式に挿入したいわゆる宇宙項で、これによって宇宙は等方で一様で静的であるという (アインシュタインの静的宇宙) 元の理論が保たれる。この定数によって遠距離における重力による引力とバランスすることができた。
- フリードマン・モデル:
Alexandre Friedmann (1922 )による。もし宇宙の物質が一定の臨界密度未満の場合は宇宙は永遠に膨張する。もしこの臨海密度超の場合は重力場は宇宙の膨張を止めて、限りなく収縮に向かう。
臨海密度はハッブル定数の2乗に比例する。なお、臨海密度は$5 \times 10^{-30}$ g/cm3で、1立米中に水素原子3個に相当。
- ハッブル図:
宇宙の拡大と収縮について。
宇宙速度が脱出速度以上かどうかはその減速を測定することでわかる。もし減速が一定数値以下ならは脱出速度以上となる。
見かけの明るさと距離(百万光年)の関係はハッブル図と呼ばれる。
>Top 3. Radiation background:
- <Bell Telephone Laboratory>
In 1964 Arno A. Penzias and Robert W. Wilson: intended to measure
the radio noise coming from our own galaxy - in effect, from the
sky itself. It is crucially important to identify any electrical
noise that might be produced within the receiving system.
They received a sizable amount of microwave noise at 7.35 cm
that was independent of direction. This static did not vary with
the time of day or with the season. It were not coming from the
Milky Way, but from a much larger volume of the universe.
- Incidentally, radio waves with wavelengths like 7.35 cm are
known as "microwave radiation", and having an equivalent
temperature of 3.5 degrees Kelvin.
- Bernard Burke of MIT and P.J.E. Peebles argued that there ought
to be a background of radio noise left over from the early universe.
- Properties of Radiation: Wavelength / Black-body K
Kind of radiation
Wavelength
Photon energy
(electron volt)
Black body
temp. (K)
Radio (-VHF)
> 100 mm
< 0.00001
< 0.03
Microwave
0.1 - 100 mm
0.00001 -
0.01
0.03 - 30
Infrared
1 - 100μm
0.01 - 1
30 - 3,000
Visible
0.3 - 1μm
1 - 6
3,000 - 15,000
Ultraviolet
1 - 300 nm
6 - 1 K
15,000 - 3 M
X ray
0.01 - 1 nm
1 K - 100 K
3 - 300 M
γray
< 0.01 nm
> 100 K
> 300 M
- Remarks: Penzias and Wilson discovered the cosmic radiation background
was 7.35 cm, which is microwave radiation.
- The photon energy released by radioactive decay is about a million
electron volts, so this is a γ ray.
- The surface of the sun is at 5,800°K, so the sun emits visible
light.
- G.D. Birkhoff Theorem: (1923):
In order to calculate the motion of any typical galaxy relative
to our own, draw a sphere with us at the center and the galaxy of
interest on the surface; the motion of this galaxy is precisely
the same as if the mass of the universe consisted only of the matter
within this sphere, with nothing outside.
- >Top Planck distribution: (by 1890s)
It had become known that the properties of radiation in a state
of thermal equilibrium with matter depend only on the temperature.
Essentially the same formula also gives the amount of radiation
emitted per second and per cm2 at any wavelength from any totally
absorbing surface, so radiation of this sort is generally known
as "black-body radiation."
- Energy of a photon with a wavelength of 1 cm:
0.000124 electron volts, and proportionally more at shorter wavelength.
(Re: 1 eV = the energy gained by 1 electron in moving
across a voltage drop of 1 volt.)
- A typical photon in visible light with wavelength of 5 x 10^-5
cm: so its energy would be 0.000124 eV x 20,000 = 2.5 eV
- The energy of a photon is very small in macroscopic terms,
which is why photons seem to blend together into continuous
stream of radiation.
- Incidentally, chemical reaction energies are generally of
the order of an eV per atom or per electron; to rip the electron
out of a hydrogen atom takes 13.6 eV.
Photons in sunlight also have energies of the order of an eV;
it is what allows these photons to produce chemical reactions
essential for life, such as photosynthesis.
- Nuclear reaction energies are generally of the order of a million eV per atomic nucleus.
- Einstein tells us that any photon's wavelength is inversely proportional
to the photon energy:
Hence, the typical wavelength of photons in black-body radiation
is inversely proportional to the temperature.
- an opaque body at an ordinary room of 300 degrees K will emit
black-body radiation with a wavelength of 0.29 cm divided by
300. (= infrared radiation)
- Surface of the sun is about 5,800 degrees K:
will emit 5,000 Angstrom (visible wavelength)
- Stefan-Boltsmann Law:
The energy density is simply the number of photons per liter times
the average energy per photon.
- The number of photons per liter is proportional to the cube
of the temperature.
- While, the average photon energy is simply proportional to
the temperature.
- Hence the energy per liter in black-body radiation is proportional
to the fourth power of the temperature.
- Black-body radiation of 3 degrees K; then its energy density
is $
4.72 \times 3^4$, or about 380 eV per liter.
3.背景放射:
- <ベル電話研究所>:
Arno A. PenziasとRobert W. Wilson (1964)は、我々の銀河、実際には空からやってくる無線雑音を測定した。これは受信システム内で発生するであろう電子ノイズを特定する上で重要であった。
彼らは7.35 cmのマイクロ波ノイズを方向に関係なく受信した。このノイズは昼夜や季節に関わりなくやってきた。それは我々の銀河からではなく、ずっと大きな宇宙からやってきた。
- 波長7.35 cmの電波はマイクロ波放射として知られ、これは絶対温度3.5度に相当。
- Bernard Burke (MIT) と P.J.E. Peeblesとはこれは初期宇宙の残りとしての背景ノイズに違いないと議論した。
- 放射の特性:波長/黒体温度K (←詳細左図)
-
電磁波の種類
黒体温度(K)
電波(-VHF):
<0.03
マイクロ波:
0.03 - 30
赤外線:
30 - 3K
可視光線:
3K - 15K
紫外線:
15K - 3M
X線:
3M - 300M
γ線:
> 300M
- 注:PenziasとWilsonが発見した
宇宙背景放射の波長7.35cmは
、マイクロ波に相当。
- γ線崩壊によって放射される光子エネルギーは百万eV。
- 太陽表面温度は5,800度Kで、太陽は可視光を放射している。
- Birkhoffの原理: (1923)
ある銀河の我々に対する相対的な動きを計算する場合は、我々を中心とし、その銀河を表面 する球を描く。その銀河の動きはあたかも宇宙の質量がこの球の中だけと見なし、外側が考慮しないで計算すればよい。
- プランクの放射法則: (1890迄)
一般に、物質の熱平衡状態における放射は温度だけに依存する。
本質的には、全ての波長を吸収する表面からのいかなる波長の毎秒単位 面積当たりの放射量についても同じ式が成り立つ。この放射が"黒体放射"である。
- 波長1cmの1光子のエネルギー:
0.000124 eVで、波長の短さに比例する。
(注:1 eV = 1Vの電位差を横切ることによって1電子の得る運動エネルギー)
- 可視光(0.5μm)のエネルギーは、約2.5eV
- 光子のエネルギはマクロ的には非常にちいさいので、放射光の流れとしては光子は連続しているように見える。
- たまたま化学反応のエネルギーは1原子あるいは1電子当たりの約1eVのレベル。
水素原子から電子を遊離させるエネルギーは13.6eV。
太陽光の光子もeVのオーダーのエネルギーを持つので、これられの光子は、光合成のような生命に必須な化学反応を作れる。
- 核反応エネルギーは原子核当たり、約100万eVのオーダー。
- アインシュタインによれば、どの光子の周波数も光子のエネルギーに反比例する。従って、黒体放射の光子周波数は温度に反比例する。
- 室温300度K(27度C)における不透明の物体は0.29 cm /300の黒体放射(赤外線に相当)をしている。
- 太陽表面は5,800度Kなので、5,000オングストローム(可視光)を放射している。
- ステファン・ボルツマンの放射法則:
エネルギー密度=光子密度 ☓ 光子当たりの平均エネルギー
- 光子密度は温度の3乗に比例
- 平均光子エネルギは温度に比例
- 従って、黒体放射におけるエネルギー密度は絶対温度の4乗に比例する。
- 3度Kの黒体放射のエネルギーは、
$
4.72 \times 3^4$ eV
=約380 eV
>Top <COBE>
- In 1989 COBE (COsmic Background Explorer)
satellite map of
temperature inhomogeneities in the cosmic microwave
background over the entire sky. Higher than average regions are shown
in red, cooler than average in blue. The total temperature range shown
in +-200 millionths of K.
- Density of nuclear particles:
In the present universe is somewhere between 6 and 0.03 particles
per thousand liters: Thus there are between 100M- 20,000M photons
for every nuclear particles.
- In the early universe, at temperature about 3,000 degrees K, the
universe consists not of the galaxies and stars we see in the sky
today, but only of an ionized and indifferentiated soup of matter
and radiation
- >Top The energy in the mass of a nuclear particle is give by Einstein's
formula $E = mc^2$ as about 939 M eV.
- "Radiation-dominated era":
At earlier times the temperature was above about 4,000 degrees
K, in which most of the energy in the universe was in the form
of radiation.
- "Matter-dominated era":
At about 3,000 degrees K, the contents of the universe were
becoming transparent to radiation.
The average energy of a photon in 3 degrees K is about 0.0007
eV, so that even with 1,000 M photons per neutron or proton,
most of the energy of the present universe is in the form of
matter, not radiation
- The reaction will still occur if the energy of the individual
photons is greater than $mc^2$; the energy will simply
go into giving the material particles a high velocity. However,
particles of mass $m$ cannot be produced in collisions
of two photons if the energy of the photon is below $mc^2$.
- Boltzmann's constant:
0.00008617 eV/degree K.
($8.617 \times 10^{-5} $ eV/K, or $1.381 \times 10^{-23}$ J/K)
- The lightest material particles are electron (e-)
and positron (e+). The positron has opposite electrical
charge but the same mass and spin. When a positron collides with
an electron, the charges can cancel, with the energy in the two
particles' masses appearing as pure radiation. The annihilation
process can also run backward - two photons with sufficient
energy can collide and produce an electron-positron pair,
the photon energies being converted into the electron and positron
masses.
- Properties of some elementary particles:
-
Particle
Symbol
Rest energy
(M eV)
Threshold temp
(B degK)
Mean life
(sec)
Photon
γ
0
0
stable
<Leptons>
weak interaction
Neutrino
νe,
ν-e
0
0
stable
νμ ,ν-μ
0
0
stable
Electron
e-,
e+
0.5110
5.930
stable
Muon
μ-,
μ+
105.66
1,226.2
2.197 x 10^-6
<Hadrons>
strong interaction
Pi meson
π
134.96
1,566.2
0.8 x 10^-16
π+,
π-
139.57
1,619.7
2.60 x 10^-8
Proton
p, p-
938.26
10,888
stable
Neutron
n, n-
939.55
10,903
920
- "Rest energy" is the energy that would be released
if all the mass of the particle were converted into energy. (million
eV)
- "Threshold temperature" is the rest energy divided
by Boltzmann's constant; it is the temperature above which a particle
can be freely created out of thermal radiation (billion degree-K)
- "Mean life" is the average length of time the
particle survives before it suffers a radioactive decay into other
particles (seconds)
- This table can tell which particles could have been present in
large numbers at various times in the history of the universe; they
are just the particles whose threshold temperature were below the
temperature of the universe at that time.
- Early universe at above the threshold of 6 billion degrees can
be considered to be composed predominantly of photons, electrons,
and positrons, not just photons alone.
<COBE>
- 1989年、COBE (宇宙背景探査衛星)による全天における宇宙背景放射(マイクロ波)の不均一な温度地図。100万分の200度K±の温度変化 (赤が高く、青が低い)(←左図参照)
- 原子核の密度:
現在の宇宙には、1立方m中に、およそ6 - 0.03個の原子がある。これは原子核1個に対し、光子の数は1億から200億の間になる。
- 初期宇宙では、温度が約3,000度Kで、宇宙は今日我々が見るように銀河や恒星から出来ていないで、イオン状態の物質と放射の未分離スープの状態であった。
- 原子核の質量にあるエネルギーはアインシュタインの公式 $E = mc^2$によって、約939M eVとなる。
- 放射優勢の時期:
初期には、温度は4,000度K以上もあり、宇宙のほとんどのエネルギーは放射の形態であった。
- 物質優勢の時期:
およそ3,000度Kでは、宇宙の中身は放射は透明となりつつあった。
3度Kにおける光子の平均エネルギーは0.0007 eVであり、中性子や陽子に対し、10億個もの光子があったとしても、現在の宇宙のほとんどのエネルギーは放射ではなく物質の形態である。
- もし光子それぞれのエネルギーが$mc^2$より大きければ反応が起こり、エネルギーが粒子に高速度を与えることになる。しかしもし光子のエネルギーが $mc^2$より小さければ2つの光子衝突によって質量 $m$は生成されない。
- ボルツマン定数:
0.00008617 eV/度K
($8.617 \times 10^{-5}$ eV/K, or $1.381 \times 10^{-23}$ J/K)
- 最軽量の素粒子は電子(e-)と陽電子(e+)である。陽電子は電荷が反対で、質量
・スピンは同じである。陽電子が電子と衝突すると電荷が消滅し、2つの粒子の質量 に相当するエネルギーが放射される。対消滅のプロセスは逆に起こり得る。十分なエネルギーを得ると2つの光子は衝突し、電子−陽電子の対を生成する。光子のエネルギーは電子と陽電子の質量
に変換される。
- 主な素粒子:(←詳細左図)
-
素粒子
記号
光子
γ
<レプトン> 弱い相互作用
ニュートリノ
νe,
ν-e
νμ ,ν-μ
電子
e-,
e+
ミュー粒子
μ-,
μ+
<ハドロン> 強い相互作用
パイ中間子
π
π+,
π-
陽子
p, p-
中性子
n, n-
- "Rest energy"(静止エネルギー)は、粒子の全質量がエネルギーに転換した場合に放出されるエネルギー(百万eV)
- "Threshold temperature"(臨海温度)は、Rest energyをボルツマン定数で割った温度(K)。この温度以上ならば粒子は熱放射から自由に生成され得る(十億度K)
- "Mean life"(平均寿命)は、粒子が放射性崩壊によって他の粒子に変化するまでの平均寿命(秒)
- この図は、どの粒子が宇宙の歴史の各段階で多く存在していたかを示す。宇宙のそれぞれの時点での臨海温度以下の粒子のみが存在できる。
- 60億度K以上の初期宇宙では、光子だけでなく、他に電子、陽電子が存在していたと考えられる。
>Top 4. Reactions in the early universe:
- Electric Charge:
We can create or destroy pairs of particles with
equal and opposite electric charge, but the net electric charge
never changes.
- Baryon Number:
Baryon includes proton, neutron and heavier unstable
particles known as hyperon. Baryons and antibaryons can be created
or destroyed in pairs; however the total number of baryons minus
the numbers of antibaryons never changes. We attribute a baryon
number of +1 to the proton, neutron and hyperon, and -1 to the corresponding
antiparticles; the rule is then that the total baryon number never
changes.
- Lepton Number:
Lepton includes light negatively charged electron
and muon, plus an electrically neutral particle of zero mass called
neutrino and their antiparticles (positron, antimuon and antineutrino).
Despite their zero mass and charge, neutrinos and antineutrinos
are no more fictitious than photons; they carry energy and momentum
like any other particle. The total number of leptons minus the total
number of antileptons never changes.
- A good example; radioactive decay of a neutron n into a proton p, an electron e-, and
an antineutrino νe-.
-
neutron
proton
electron
antineutrino
n →
p +
e-
νe-
Charge
0
+1
-1
0
Baryon #
+1
+1
0
0
Lepton #
0
0
+1
-1
4.初期宇宙における反応:
- 電荷:
粒子を等しい正負の電荷をもつ粒子に作ったり壊したり出来るが、電気量 の和は不変である。(電荷保存則)
- バリオン数 (重粒子数):
バリオンとは陽子、中性子や重たく不安定なハイペロンと呼ばれる素粒子をいう。バリオンと反バリオンとは対になって作られあるいは壊される。陽子、中性子、ハイペロンをバリオン数+1とし、対応する反粒子を-1とすると、バリオン数は不変である。
- レプトン数 (軽粒子数):
レプトンとは、負電荷をもつ電子やミューオン、および電荷をもたないゼロ質量 のニュートリノおよびこれらの反素粒子(陽電子、反ミューオン、反ニュートリノをいう。質量
もゼロ、電荷もゼロというニュートリノや反ニュートリノは光子より架空という訳ではない、それは他の素粒子同様にエネルギーと運動量 をもつ。レプトンの合計数量と反レプトンの合計数量との差は不変である。
- わかりやすい事例として、中性子nが放射性崩壊をして、陽子pと電子e-と反ニュートリノνe-になる。
(←左図参照)
>Top 5. The first Three Minutes:
- I will adjust the speed of our film to the falling temperature of
the universe. Unfortunately, I cannot start the film at zero time
and infinite temperature.
- <I cannot start the film at zero time>
$1.5 \times 10^{12}$ degrees K; 0.01 seconds; the universe contains
large number of pi mesons, which weigh about 1/7 as much as a nuclear
particle.
Pi mesons interact very strongly with each other and with nuclear
particles.
Quark theory suggests that around several million million degrees
K, the hadrons would simply break up into their constituent quarks,
just as atoms break up into electrons and nuclei at a few thousand
degree, and nuclei break up into protons and neutrons at a few thousand
million degrees. The universe could be considered to consist of
photons, leptons, antileptons, quarks, and antiquarks, all moving
essentially as free particles.
(But the puzzle of the nonexistence of isolated free quarks is
one of the most important problems facing theoretical physics the
the present moment.)
- <First Frame>
$10^{11}$ degrees K; above threshold temperature for electrons
and positrons:
Despite its rapid expansion, the universe is nearly perfect thermal
equilibrium. The universe is so dense that even the neutrinos (here
means neutrinos and antinueutrinos) are kept in thermal equilibrium
with the electrons, positrons, and photons by rapid collisions with
them and with each other.
The total energy density of the universe at this temperature is
$
21\times 10^{44}$ eV per liter, which is equivalent to a mass density of
3.8 billion kg/liter.
The most important reactions are:
- Antineutrino (νe-) plus proton (p) yields positron (e+) plus neutron
(n) (and vice versa)
- Neutrino (νe) plus neutron (n) yields electron
(e) plus proton (p) (and vice versa)
- How large the universe was at very early times?
Since the temperature of the universe falls in inverse proportion
to its size; this gives a circumference of about four light
years.
- <Second Frame>
$3 \times 10^{10}$ degrees K; 0.11 seconds have elapsed.
Nothing has changed qualitatively - the contents are still dominated
by electrons, positrons, neutrinos, antineutrinos, and photons,
all in thermal equilibrium.
The small number of nuclear particles is still not bound into nuclei,
but with falling temperature the heavier neutrons to turn into the
lighter protons than vice versa. 38% neutrons and 62% protons.
- <Third Frame>
$10^{10}$ degrees K; 1.09 seconds have elapsed. Decreasing
density and temperature have increased free particle of neutrinos
and antineutrinos, no longer in thermal equilibrium with electrons,
positrons or photons. ("decoupling")
The total energy density decreases, now equivalent to mass density
380 K times that of water. Now proton-neutron balance; 24% neutrons
and 76% protons.
- <Fourth Frame>
$3 \times 10^9$ degrees K; 13.82 seconds have elapsed. Now below
the threshold temperature for electrons and positrons, so rapidly
to disappear as major constituents of the universe. From now on,
the temperature of the universe means that of photons.
A proton and a neutron can form a deuterium with extra energy and
momentum being carried away by a photon. The deuterium nucleus can
then collide with a proton or a neutron, forming helium three (He3, two protons and a neutron), or tritium (H3, a proton and two neutrons). Finally H3 collide
with a neutron and H3 collide with a proton, forming ordinary helium
(He4, two protons and two neutrons). The
balance now is 17% neutrons and 83% protons.
- <Fifth Frame>
$10^9$ degrees K, 3 minutes and 2 seconds have elapsed.
The electrons and positrons have mostly disappeared, and the chief
constituents are now photons, neutrinos and antineutrinos. The universe
is now cool enough for H3 and He3 as well as ordinary He nuclei
to hold together. The decay of free neutron is beginning to be important;
the balance is now 14% neutrons, 86% protons.
5.最初の3分間:
- ここでは、フィルムのスピードを宇宙の温度の降下に合わせて調整してみよう。但しそのフィルムはゼロ時間および無限大の温度から始まってはいない。
- <ゼロ時間から始まっていない>
$1.5 \times 10^{12}$ 度K, 0.01秒後。宇宙には核子の1/7の質量をもつパイ中間子が大量 に存在している。パイ中間子は相互にまた核子と強く相互作用している。
クォーク理論によれば数$10^{12}$度Kの辺りでハドロンはクォークに分解する。あたかも数鮮度で原子が電子と原子核に分解したり、また数十億度Kで原子核が陽子と中性子に分解するのと同様である。宇宙は、光子・レプトン・反レプトン・クォーク・反クォークがすべて自由素粒子として動き回っている。
(但し、今日まで孤立した自由クォークの存在が確かめられていないのは理論物理の最重要課題の一つである。)
- <1コマ目>
$10^{11}$ 度K、電子と反電子の閾値温度以上。
急激な宇宙の膨張にもかかわらず、ほぼ完全な熱平衡状態にある。宇宙は濃密でニュートリノ(ここではニュートリノおよび反ニュートリノをまとめていう)は電子、反電子、光子とそれぞれ、相互に衝突し、熱平衡状態にある。
この温度における宇宙のエネルギー密度は
$
21\times 10^{44}$ eV/リットルで、これは38億kg/リットルに相当する。
重要は反応としては、
- 反ニュートリノ(νe-)と 陽子(p)
によって、陽電子(e+)と中性子(n) を生成。
(その逆もあり)
- ニュートリノ(νe)と中性子 (n)によって、電子(e)と陽子(p)を生成。
(その逆もあり)
- 初期宇宙の大きさは?
宇宙の温度は、その大きさに逆比例する。およそ4光年。
- <2コマ目>
$3 \times 10^{10}$度K; 0.11秒経過
質的には変化なし。中身は電子、陽電子、ニュートリノ、反ニュートリノ、光子からなり、熱平衡状態にある。少量 の各紙はまだ核にしばりつけられていないが、温度降下に伴いより重い中性子は軽い陽子に変化したり、またその逆もある。その比率は中性子38%に対し陽子は62%。
- <3コマ目>
$10^{10}$度K; 1.09秒経過。
密度と温度の降下に伴い、自由ニュートリノおよび反ニュートリノが増加し、電子、陽電子、光子の熱平衡が崩れ出す。(分離)
全エネルギー密度は減少し、水の密度の38万倍程度になる。電子・中性子バランスは、中性子24%に対し陽子は76%。
- <4コマ目>
$3 \times 10^9$ 度K; 13.82秒経過。
電子および陽電子の閾値温度以下となり、宇宙の主要構成物質ではなくなる。以後は、宇宙の温度は光子の温度となる。
陽子と中性子によって、重水素と余剰エネルギーと運動量をもつ光子が生成する。さらに重水素は陽子または中性子と衝突し、ヘリウム3 (2陽子と1中性子)または三重水素 (1陽子と2中性子)を生成する。最後に三重水素が中性子と、また陽子と衝突しヘリウムを生成する。バランスは中性子17%に対し陽子は83%。
- <5コマ目>
$10^9$ 度K, 3分2秒経過。
電子と陽電子はほぼ消滅し、主な抗生物質は光子、ニュートリノ、反ニュートリノとなる。宇宙は三重水素、ヘリウム3、ヘリウムが存在できるまでに冷却。自由中性子の崩壊が重要になりつつある。バランスは中性子14%に対し陽子は86%。
- >Top
- <A Little Later>
$0.9 \times 10^9 $ degrees K, 3 minutes and 46 seconds passed.
A dramatic event occurs; the temperature drops to the point at which
deuterium nuclei can hold together; almost all of the remaining
neutrons are immediately cooked into helium nuclei.
The balance is 13% neutrons, 87% protons.
- <Sixth Frame>
$3 \times 10^8$ degrees K, 34 minutes and 40 seconds passed.
The electrons and positrons are now completely annihilated except
for the small excess of electrons needed to balance the charge of
the protons.
Nuclear processes have stopped - now mostly bound into helium nuclei
or free protons, with about 22 - 28% helium by weight.
- <After 700,000 years>
The universe will go on expanding and cooling, where electrons and
nuclei can form stable atoms; the lack of free electrons will make
the contents of the universe transparent to radiation; and the decoupling
of matter and radiation will allow matter to begin to form into
galaxies and stars.
- <After another 10 billion years>
Living beings will begin to reconstruct this story.
In addition to the large amount of helium produced at the end of
the first 3 minutes, there was also a trace of deuterium and light
helium isotope He3. The deuterium abundance is very sensitive to
the density of nuclear particles at the time of nucleosysthesis:
if 20 ppm of deuterium was really created in the early universe,
then there must have been (and is now) just about 1.1 billion photons
per nuclear particle.
- I do not believe that scientific progress is always best advanced
by keeping an altogether open mind. The great thing is not to be
free of theoretical prejudices, but to have the right theoretical
prejudices. The standard model of the early universe has some success,
and it provides a coherent theoretical framework for future experimental
programs.
- <その少し後>
$0.9 \times 10^9 $度K, 3分46秒経過
劇的な変化が起こる。温度は重水素の原子核が融合できる程度にまで降下し、残存していた中性子はほとんどヘリウムの原子核に取り込まれる。バランスは中性子13%に対し陽子は87%。
- <6コマ目>
$3 \times 10^8$ 度K, 34分40秒経過 電子と陽電子は、陽子の電荷に相当する僅かの過剰な電子を残し、完全に消滅。核反応は停止し、ほとんどがヘリウムの原子核または自由陽子に取り込まれる。ヘリウムは重量
比で22-28%を占める。
- <70万年後>
宇宙は膨張し、冷却し続け、電子と原子核は安定的な原子を構成する。自由電子がなくなったことで宇宙の構成物質は放射に対し透明になり、また物質と放射の分離によって物質は銀河や構成を生成し始める。
- <100億年後>
生命がこの物語を再編し始める。
最初の3分間で大量のヘリウムが生成され、また僅かな重水素やヘリウム3同位 体も残存した。重水素の量は核合成の時期における核子の濃度に大きく関係する。もし初期宇宙で20
ppmの重水素が生成されたとすれば、核子当たり11億の光子が生成されたことになる。
- 科学の進歩は常にオープンマインドであればいいというものではないと思う。重要なことは、理論的な偏見から自由でないということで、むしろ正しい理論的偏見を持つべきである。初期宇宙における標準モデルがある程度成功し、将来の実験計画について一貫した理論的枠組みを与えている。
>Top 6. Epilogue:
- The UNIVERSE will certainly go on expanding for a while; the standard
model gives an equivocal prophecy: It all depends on whether the cosmic
density is less or greater than a certain critical value.
- If the cosmic density is less than the critical
density:
Our descendants will see thermonuclear reactions slowly come to
an end in all the stars, leaving behind various sorts of cinder;
black dwarf stars, neutron stars, perhaps black holes. The cosmic
backgrounds of radiation and neutrinos will continue to fall in
temperature.
- If the cosmic density is greater than the critical
density; then the universe is finite and its expansion will eventually
cease, giving way to an accelerating contraction.
- If the cosmic density is twice its critical value, then the
universe is now 10 billion years old; it will go on expanding
for another 50 billion years, and then begin to contract. After
50 billion years the universe would have regained its present
size, and after another 10 billion years it would approach a
singular state of infinite density. During the early part of
the contracting phase, astronomers will be able to observe both
red shifts (from distant galaxies) and blue shifts (from nearby
objects) .
- As the universe begins to contract, the temperature will start
to rise. When the universe has recontracted to 1/100 its present
size, the radiation background will begin to dominate the sky:
the night sky will be as warm (300 degrees K) as our present
sky at day.
- 70 million years later the universe will have contracted
another tenfold, and our heirs will find the sky intolerable
bright. Molecules in planetary and stellar atmospheres and in
interstellar space will begin to dissociate into their constituent
atoms, and the atoms will break up into free electrons and atomic
nuclei.
- After another 700,000 years, the cosmic temperature
will be at ten million degrees; then stars and planets will
dissolve into a cosmic soup of radiation, electrons, and nuclei.
- In another 22 days, the nuclei will then begin to break
up into their constituent protons and neutrons. soon after that,
electrons and positrons will be created in great numbers in
photon-photon collisions, and the cosmic background of neutrinos
and antineutrinos will regain thermal communion with the rest
of the universe.
- <Oscillating model>
If this is our future, it presumably also is our past. The present
expanding universe would be only the phase following the last contraction
and bounce. It is assumed that there was a previous complete phase
of cosmic expansion and contraction. Such oscillating model, like
the steady-stage model, nicely avoids the problem of Genesis.
- However, in each cycle the ratio of photons to nuclear particle
is slightly increased by a kind of friction as the universe
expands and contracts. As far as we know, the universe would
then start each new cycle with a new, slightly larger ratio
of photons to nuclear particles. So it is hard to see how the
universe could have previously experienced an infinite number
of cycles.
- It is almost irresistible for humans to believe that we have
some special relation to the universe, that human life is not just
a more-or-less farcical outcome of a chain of accidents reaching
back to the first three minutes, but that we were somehow build
in from the beginning.
6. 結語:
- この宇宙は、しばらくは確実に膨張し続ける。その後の予言については、標準モデルではあいまいである。すべては宇宙密度が一定の臨界量 以下かそれ以上かにかかっている。
- もし、宇宙密度が臨海密度以下の場合は、我々の子孫はすべての恒星における熱核反応が徐々に終わり、暗い矮星、中性子星、あるいはブラックホールを残す。宇宙の背景放射とニュートリノは引き続き温度低下を示す。
- もし宇宙密度が臨海密度より大きい場合は、宇宙は有限でその膨張はいずれ終了し、収縮を開始することになる。
- もし宇宙密度が臨海密度の2倍とすると、宇宙は今100億年なのであと500億年は膨張し続け、それから収縮し始める。500億年後に宇宙は再び現在の大きさに戻り、その後は100億年かけて無限大の密度の特異点に近づく。収縮の初期段階では、天文学者は赤方偏移(遠方の銀河)と青方偏移(近くの物体)の両方が観測できる。
- 宇宙が収縮を始めると、温度は上昇に転じる。宇宙が現在の1/100に収縮すると、背景放射は空を覆い、夜空でも300度Kになり昼間のように輝く。
- 7千万年後は宇宙はさらに10倍に収縮し、我々の子孫は空が耐えられないように明るく輝くのを見るだろう。惑星や恒星の大気や恒星間の分子はそれらを構成する原子に分解し、さらに原子は自由電子と原子核に分解。
- さらに70万年後には、宇宙温度は1000万度となり、恒星や惑星は放射、電子、原子核からなる宇宙のスープ状に分解。
- さらに22日後、原子核は、陽子や中性子に分解し、間もなく電子や陽電子が膨大な量 の光子同士の衝突によって生成される。宇宙の背景はニュートリノと反ニュートリノによって再び宇宙全体が満たされる。
- <振動モデル>
上記は我々の未来であるならば、それを我々の過去とみなすこともできる。現在の膨張宇宙は過去の収縮およびその反転の結果かもしれない。過去においては宇宙の膨張と収縮を完全に繰り返すと想定するという説がある。この振動モデルは、静止モデルと同様に宇宙創生問題をうまく避けることができる。
- しかしながら、各サイクル毎に光子の核子に対する比率は摩擦のように宇宙の膨張と収縮に伴い若干増加する。我々の知る限り、光子の核子に対する比率はほとんど増えないので、宇宙はまった新しい状態からサイクルを開始するように見える。従って宇宙が過去に無限回のサイクルを経験したとは見なせない。
- 人類が宇宙に特別な関係をもっている、即ち、人類の生命は、宇宙創生の最初の3分間に起こった偶然以来の連鎖の滑稽な結末ではなく、最初からそうなるべくしてなったのだと信じたいという考えにほとんどは抗しがたいものがある。
Comment
- Since my childhood, there is the basic question: "Where are
we from, and where are we going?" There has been no satisfactory
answers to this for a long time. But this book seems to describe
closer answers to this.
- In 21st. century, we must continue to pursue the answer to such
basic questions - about universe, about life, about our future and
about our raisons d'etre.
- 子供の頃からの基本的な質問がある。「我々はどこから来て、どこへ行こうとしているのか?」長い間これに対する満足する答えがなかった。しかしこの本はそれに近い答えが書いてあるような気がする。
- 21世紀には、これらの基本的な質問に対する答えを引き続き探し続けなければならない。我々の宇宙・生命・未来そして存在意義について...
![]() |
The First Three MinutesA Modern View of the Origin of the Universe |
Cat: SCI
|
Steven Weinberg |
93131u/18221r |
Title |
The First Three Minutes |
宇宙創生最初の3分間 |
---|---|---|
Sub Title |
|
|
Author |
|
|
Published |
|
|
|
||
Tag |
; 1 billion K after 3min; Black body radiation; Cepheid variable; Early universe; Isotropic & homogenoius; Hubble constant; Light neutrino antineutrino; Matter dominated; Radiation background; Radiation dominated; Violent explosion; ; | |
Why |
|
|
Summary |
要約 |
||||||||||||||||||||||||||||||||||||||||||||||||||||||||||||||||||||||||||||||||
>Top 1. The Origin of the Universe:
>Top <Summary of the history of the early universe>:
|
1.宇宙の起源:
<初期宇宙の歴史のまとめ>:
|
||||||||||||||||||||||||||||||||||||||||||||||||||||||||||||||||||||||||||||||||
Future of the universe:
|
宇宙の将来:
|
||||||||||||||||||||||||||||||||||||||||||||||||||||||||||||||||||||||||||||||||
>Top 2. The expansion of the universe:
|
2.宇宙の膨張:
|
||||||||||||||||||||||||||||||||||||||||||||||||||||||||||||||||||||||||||||||||
>Top <Steps to know the expansion of the universe>
|
<宇宙膨張を知るための基礎>
|
||||||||||||||||||||||||||||||||||||||||||||||||||||||||||||||||||||||||||||||||
>Top 3. Radiation background:
|
3.背景放射:
|
||||||||||||||||||||||||||||||||||||||||||||||||||||||||||||||||||||||||||||||||
>Top <COBE>
|
<COBE>
|
||||||||||||||||||||||||||||||||||||||||||||||||||||||||||||||||||||||||||||||||
>Top 4. Reactions in the early universe:
|
4.初期宇宙における反応:
|
||||||||||||||||||||||||||||||||||||||||||||||||||||||||||||||||||||||||||||||||
>Top 5. The first Three Minutes:
|
5.最初の3分間:
|
||||||||||||||||||||||||||||||||||||||||||||||||||||||||||||||||||||||||||||||||
|
|
||||||||||||||||||||||||||||||||||||||||||||||||||||||||||||||||||||||||||||||||
>Top 6. Epilogue:
|
6. 結語:
|
Comment |
|
|
---|