Origins of the elements in the universe
Cat: SCI
Pub: 2020
#2015a
Chiaki Kobayashi, et.al.
20923u/1710r
Original resume
Remarks
>Top 0. Preface:
- It has been a long mystery to understand how each element of the periodic table is synthesized; recent researches of the galactic evolutions are finding new theory of the formation, revising the hitherto theories.
0. 序文:
- 周期律表にある各元素が、宇宙開闢以来、どのように生成されてきたのかが、長い間天文学上に謎だったが、銀河系の進化を巡る最近の研究で、従来の通説では不十分で、それらがかなり明らかになりつつある。
>Top 1. University of Hertfordshire (UK), UH, , 16 Sept 2020
- A new study of galaxy evolution, published in The Astrophysics Journal, reveals unexplained quantities of gold in the cosmos, posing an astronomical mystery. Researchers working on the project say their findings will substantially change the presently accepted view of how the universe evolved.
- >Top An international team of female astrophysics researchers led by Dr Chiaki Kobayashi, reader in Astrophysics at the University of Hertfordshire, analysed existing data in the first study of its kind to explore the origins of all the elements in the periodic table.
- Gold, along with other heavier elements, were thought to have been crated when neutron stars – the remains of highly dense burnt-out stars – crashed into one another. However, the research team found that the role of neutron stars mergers may have been considerably overestimated. It appears that another stellar explosion altogether is responsible for making most of the gold in the cosmos.
- >Top Dr Chiaki Kobayashi, University of Hertfordshire, explains: ‘Even the most optimistic estimates of neutron star collision frequency simply can’t account for the sheer abundance of gold and other elements in the Universe. This was a surprise. It looks like spinning supernovae with strong magnetic fields could be the real source of the majority of these elements. The astrophysical problem of the missing gold may be solved by a nuclear physics experiment. Research currently being carried out from nuclear facilities around the world, including Europe, the USA and Japan, with new discoveries expected.
- The international team of all female researchers has produced a new-look Periodic Table to demonstrate their findings. The team was led by Chiaki Kobayashi and included Amanda Karakas, from Australia’s Monash University and ARC Centre of Excellence for All Sky Astrophysics in 3 Dimensions (ASTRO 3D), and Dr Maria Lugaro, from Hungary’s Konkoly Observatory.
1. University of Hertfordshire (UK):
- Asymptotic giant branch stars: AGB星, 漸近巨星分枝星, 3-8倍⦿質量の恒星進化の最終段階で、中心核のC/Oが縮退し、その外側でHe/Hが核融合を起こしている星
- Ia型超新星: 白色矮星の爆発の結果生じる激変星の一つ。核がC/Oが燃焼温度に達すると、核融合が開始され数秒の内に熱暴走で崩壊し、超新星爆発を起こす。
- 周期律表上の元素毎の生成過程:
ビッグバン核融合(黒)、 AGB星(緑)、中心核崩壊超新星(青)、Ia型超新星 (赤)、中性子星合体(ピンク)
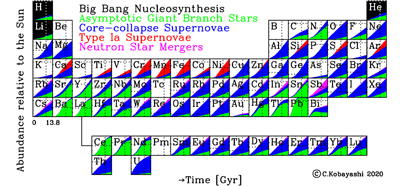
- The Astrophysics Journal誌に掲載された銀河進化の新たな研究は、いままで宇宙の謎として説明がつかなかった宇宙における金の存在量を明らかにした。研究者達にこのプロジェクトによる発見は、現在宇宙がどう進化してきたのかの通説を変えることになろう。
- Dr. Chiaki Kobayashi (University of Heretfordshireの宇宙物理学リーダー)に率いられた国際女性宇宙物理学者の研究グループは、既存のデータを解析して、周期律表の全ての元素の起源を始めて明らかにした。
- 金などの鉄より重たい元素は、恒星の最後に残る超高密度の中性子星の衝突合体によって作られると思われてきた。しかし、研究チームは、中性子星の合体は課題評価され過ぎていることを発見した。それ以外の恒星爆発によっても宇宙における金の大半を生成できることを示したのだ。
- Dr. Chiaki Kobyashiが説明するには、'中性子星衝突頻度の最も楽観的な予測であっても宇宙における金その他の原則の存在比を説明できない。このことは驚きである。おそらく強力な磁場を持つ回転している中心性がこれら元素の大半の起源となろう。'とのこと。天文学上の消えた金の問題は、核物理学実験によって検証されるだろう。現在欧州、米国、日本など世界中の核融合施設で研究がなされており、新発見が期待される。
- この国際研究者チームは、この発見によって周期律表に新たな見方を提供した。この研究チームは、Chiaki Kobayashiリーダーの下に, amanda Karakas (豪州 ASTRO 3D)、およびDr. Maria Lugaro (ハンガリーのKonkoly Observatory)から成る。
>Top 2. Science Alert: Michelle Starr, 15 Sept. 2020
- The True Origins of Gold in our Universe May Have Just Changed, Again:
- When humanity finally detected the collision between two neutron stars in 2017, we confirmed a long-held theory - in the energetic fires of these incredible explosions, elements heavier than iron are forged. And so, we thought we had an answer to the question of how these elements - including gold - propagated throughout the Universe.
- But a new analysis has revealed a problem. According to new galactic chemical evolution models, neutron star collisions don't even come close to producing the abundances of heavy elements found in the Milky Way galaxy today. "Neutron star mergers did not produce enough heavy elements in the early life of the Universe, and they still don't now, 14 billion years later," said astrophysicist Amanda Karakas of Monash University and the ARC Centre of Excellence for All Sky Astrophysics in 3 Dimensions (ASTRO 3D) in Australia.
"The Universe didn't make them fast enough to account for their presence in very ancient stars, and, overall, there are simply not enough collisions going on to account for the abundance of these elements around today." Stars are the forges that produce most of the elements in the Universe. In the early Universe, after the primordial quark soup cooled enough to coalesce into matter, it formed hydrogen and helium - still the two most abundant elements in the Universe.
The first stars formed as gravity pulled together clumps of these materials. In the nuclear fusion furnaces of their cores, these stars forged hydrogen into helium; then helium into carbon; and so on, fusing heavier and heavier elements as they run out of lighter ones until iron is produced. Iron itself can fuse, but it consumes huge amounts of energy - more than such fusion produces - so an iron core is the end point.
- >Top "We can think of stars as giant pressure cookers where new elements are created," Karakas said. "The reactions that make these elements also provide the energy that keeps stars shining bright for billions of years. As stars age, they produce heavier and heavier elements as their insides heat up." To create elements heavier than iron - such as gold, silver, thorium and uranium - the rapid neutron-capture process, or r-process, is required. This can take place in really energetic explosions, which generate a series of nuclear reactions in which atomic nuclei collide with neutrons to synthesise elements heavier than iron.
But it needs to happen really quickly, so that radioactive decay doesn't have time to occur before more neutrons are added to the nucleus.
- We know now that the kilonova explosion generated by a neutron star collision is an energetic-enough environment for the r-process to take place. That's not under dispute. But, in order to produce the quantities of these heavier elements we observe, we'd need a minimum frequency of neutron star collisions.
To figure out the sources of these elements, the researchers constructed galactic chemical evolution models for all stable elements from carbon to uranium, using the most up-to-date astrophysical observations and chemical abundances in the Milky Way available. They included theoretical nucleosynthesis yields and event rates.
- >Top They laid out their work in a periodic table that shows the origins of the elements they modelled. And, among their findings, they found the neutron star collision frequency lacking, from the early Universe to now. Instead, they believe that a type of supernova could be responsible.
These are called magnetorotational supernovae, and they occur when the core of a massive, fast-spinning star with a strong magnetic field collapses. These are also thought to be energetic enough for the r-process to take place. If a small percentage of supernovae of stars between 25 and 50 solar masses are magnetorotational, that could make up the difference.
"Even the most optimistic estimates of neutron star collision frequency simply can't account for the sheer abundance of these elements in the Universe," said Karakas. "This was a surprise. It looks like spinning supernovae with strong magnetic fields are the real source of most of these elements."
- Previous research has found a type of supernova called a collapsar supernova can also produce heavy elements. This is when a rapidly rotating star over 30 solar masses goes supernova before collapsing down into a black hole. These are thought to be much rarer than neutron star collisions, but they could be a contributor - it matches neatly with the team's other findings.
- They found that stars less massive than about eight solar masses produce carbon, nitrogen, fluorine, and about half of all the elements heavier than iron. Stars more massive than eight solar masses produce most of the oxygen and calcium needed for life, as well as most of the rest of the elements between carbon and iron.
- "Apart from hydrogen, there is no single element that can be formed only by one type of star," explained astrophysicist Chiaki Kobayashi of the University of Hertfordshire in the UK.
"Half of carbon is produced from dying low-mass stars, but the other half comes from supernovae. And half the iron comes from normal supernovae of massive stars, but the other half needs another form, known as Type Ia supernovae. These are produced in binary systems of low mass stars."
platinum traced back to a neutron star collision 4.6 billion years ago has a different origin story.
This doesn't necessarily mean that the estimated 0.3 percent of Earth's gold and platinum traced back to a neutron star collision 4.6 billion years ago has a different origin story. It's just not necessarily the whole story.
But we've only been detecting gravitational waves for five years. It could be, as our equipment and techniques improve, that we find neutron star collisions are much more frequent than we think they are at this current time.
Curiously, the researchers' models also turned out more silver than observed, and less gold. That suggests something needs to be tweaked. Perhaps it's the calculations. Or perhaps there are some aspects of stellar nucleosynthesis that we are yet to understand.
The research has been published in The Astrophysical Journal. (The American Astronomical Society)
2. Science Alert:誌の記事:
- coalesce: 合体、結合, come together to form one mass
- r-process: r (rapid)過程; 中性子星密度が高い状態で、鉄より重たい元素を生成する過程。
- kilonova: キロノヴァ。中性子星とBlack holeの合体などでr過程が発生
- collapsar: 崩壊星
- tweak:微調整
- Periodic Table
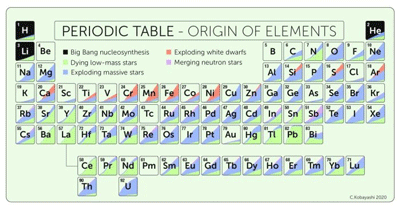
- 宇宙における金の起源は変化した:
- 2017年に2つの中性子星の衝突を観測し、長い間の理論であったこの衝突のエネルギーによって鉄より重たい元素が生成されたことを確認した。金を含むこれらの元素はこうして宇宙に拡散したと考えた。
- しかし、分析結果、新たな化学進化モデルによれば、中性子星の衝突では、今日銀河系に存在するだけの重たい元素の存在比を説明できないという。"中性子星の合体では初期宇宙での重たい元素が生成されず、それは140億年後の今日でも生成されていない。"Amanada Karakas (Monas University, ASTRO 3D豪州)は言う。"古い恒星での重たい元素存在比を十分説明できないし、今日の存在比を説明できるほど頻繁な衝突合体があったとは言えない。" 恒星は宇宙におけるほとんどの元素を生成する。初期宇宙では、クォークスープが冷却して物質が合成されて、宇宙で最も存在比が大きい水素とヘリウムができる。最初の恒星はこれらの物質の塊を重力で引きつけることで生成された。その内部での核融合によって、水素からヘリウムへ、そしてヘリウムから炭素へと次々と鉄に至るまでの重たい元素が生成される。鉄自身も核融合され得るが、それにはそれもでの核融合よりも巨大なエネルギーを必要とするので、鉄の生成で終わる。
- " 恒星は、新たな元素が生成される巨大な圧力鍋のようなものだ"と、Karakasは言う。"この核融合反応によって恒星は何十億年も輝き続けることができる。恒星が年をとると内部が高熱となりより重たい元素が生成される。" 鉄より重たい金、銀、トリウム、ウランなどを生成するには、Rapid process (r-process)が必要となる。これはエネルギー爆発の中で発生し、原子核が、中性子核融合反応が続々と衝突することで鉄より重たい原子を生成する。しかし、これは迅速に起こるので、原子崩壊する前に中性子がさらに次々と核に加えられる。
- これらの元素の原因を追求するために、研究者達は、最新の宇宙物理学の観測や銀河系の化学的存在比を使って、炭素からウランまでの安定的な全ての元素について、理論的な核融合エネルギーや現象頻度を含めた銀河系の化学進化モデルを作り上げた。
- その結果、周期律表上の元素生成のモデルを作った。さらに初期宇宙から今日まで中性子星の衝突頻度が足らず、超新星の型が関係していると結論づけた。これは磁場回転型超新星と呼ばれ、強い磁場を持ち、コアの重量が大きく、高速回転している恒星が崩壊する時に生じる。またこの時は、rプロセスが起きるほどの高エネルギー持つと考えられる。太陽質量の25-50倍の質量がある一部の超新星なら、このような違いが生じる。
"宇宙での中性子星の衝突頻度をかなり楽観的に見積もったとしても宇宙におけるこれらの元素の存在比を説明できない"と、Karakasは言う。"これは驚くべきことで、強力な磁場を持った回転する超新星が、これらの元素の供給源ということだろう。"
- それまでの研究では、超新星の崩壊によって重たい元素も生成されるとしていた。太陽質量の30倍以上もある高速回転の恒星は、ブラックホールへの崩壊する前には超新星となる。これは中性子星衝突より遥かに稀な出来事とは言え、その他の観測事実はよく説明できる。太陽質量の約8倍未満の場合は、炭素、窒素、フッ素など鉄より重い半数のが生成されるが、太陽質量の8倍より重たい星の場合は、主に酸素とカルシウム、さらに炭素から鉄までのほぼ全ての元素が生成される。
- "水素を別とすれば、どの元素も一つの型の恒星から生成される訳ではない"と小林千晶 (英国Hertfordshire University)は言う。"半数の炭素は、崩壊する低質量の星から、残りの半数は超新星から生成される。そして半数の鉄は重たい星の通常の超新星から、残りの鉄は、Ia 型超新星 (低重量の連星から起こる)から生成される。" 46億年前の中性子星衝突で生成した白金については、別の話であって、これは地球上にある金と白金の0.3%が、46億年前の中性子星衝突に起因している。我々が重力波を観測できたのはここ5年間である。もっと観測機械や技術が向上すれば、中性子星の衝突は、現在より頻繁に観測できるようになる。
また奇妙なことに、研究者達のモデルでは、銀の生成は、存在比より大きくなり、金については少なくなるので、モデルの微調整は必要となろうが、それより、恒星の核融合についてはまだ不明のことがあるという。
この研究は、The Astreophysical Journal (米国天文学会)で発表された。
>Top 3. The Daily Galaxy, Great Discoveries Channel, Posted on Sep. 15, 2020
- Sunlight is the most precious gold on Earth, biologists say. But for astronomers;
- the search for the source of the amount of gold existing in in the cosmos continues despite recent hypotheses that most of the precious metal was created by neutron star collisions. On 17 August 2017, the first-ever observations of the gravitational wave signal lasting for about 100 seconds by the LIGO and Virgo detector of two colliding neutron stars stunned the world’s astronomy community.
- The event, labeled GW170817, sent gravitational waves rippling through space that were detected by orbiting and ground-based telescopes around the world. It was the first event ever to be detected both by gravitational waves and electromagnetic waves, including gamma rays, X-rays, visible light, and radio waves
- Mystery of the Missing Gold:
- A new analysis of galaxy evolution finds that the stellar collisions do not create the quantity of chemical elements previously assumed. The research also reveals that current models can’t explain the amount of gold in the cosmos—creating an astronomical mystery. The work has produced a new-look Periodic Table showing the stellar origins of naturally occurring elements from carbon to uranium, with gold still a question mark.
- All the hydrogen in the universe—including every molecule of it on Earth—was created in the Big Bang, which also produced a lot of helium and lithium, but not much else. The rest of the naturally occurring elements are made by nuclear processes happening inside stars. Mass governs exactly which elements are forged, but they are all released into galaxies in each star’s final moments—explosively, in the case of really big ones, or as dense outflows, similar to solar wind, for ones in the same class as the sun.
- Stars as Giant Pressure Cookers:
- “We can think of stars as giant pressure cookers where new elements are created,” explained co-author Associate Professor Amanda Karakas from Australia’s ARC Center of Excellence for All Sky Astrophysics in Three Dimensions (ASTRO 3-D). “The reactions that make these elements also provide the energy that keeps stars shining brightly for billions of years. As stars age, they produce heavier and heavier elements as their insides heat up.”
- Dark Hearts of the Cosmos - Dazzling New Mergers of Black Holes and Neutron Stars:
- Gravitational-wave researchers increasingly think that globular clusters, dazzling, celestial “snow globes” populated with hundreds of thousands of closely packed stars, harbor dark “hearts,” loaded with dozens to even hundreds of black holes–by far the greatest concentration of these exotic objects found anywhere in the universe. Their hunches appear to have paid off, reports
- Undiscovered Stellar Processes:
- Half of all the elements that are heavier than iron—such as thorium and uranium—were thought to be made when neutron stars, the superdense remains of burnt-out suns, crashed into one another. Long theorized, neutron star collisions were not confirmed until 2017. Now, however, fresh analysis by Karakas and fellow astronomers Chiaki Kobayashi and Maria Lugaro reveals that the role of neutron stars may have been considerably overestimated—and that another stellar process altogether is responsible for making most of the heavy elements.
- “Neutron star mergers did not produce enough heavy elements in the early life of the universe, and they still don’t now, 14 billion years later,” said Karakas. “The universe didn’t make them fast enough to account for their presence in very ancient stars, and, overall, there are simply not enough collisions going on to account for the abundance of these elements around today.”
- Instead, the researchers found that heavy elements needed to be created by an entirely different sort of stellar phenomenon—unusual supernovae that collapse while spinning at high speed and generating strong magnetic fields. The finding is one of several to emerge from their research, which has just been published in the Astrophysical Journal. Their study is the first time that the stellar origins of all naturally occurring elements from carbon to uranium have been calculated from first principles.
- The New Modeling:
- The new modeling, the researchers say, will substantially change the presently accepted model of how the universe evolved.”For example, we built this new model to explain all elements at once, and found enough silver but not enough gold,” said co-author Kobayashi, from the University of Hertfordshire in the UK.
- “Silver is over-produced but gold is under-produced in the model compared with observations. This means that we might need to identify a new type of stellar explosion or nuclear reaction.” The study refines previous studies that calculate the relative roles of star mass, age and arrangement in the production of elements. For instance, the researchers established that stars smaller than about eight times the mass of the sun produce carbon, nitrogen and fluorine, as well as half of all the elements heavier than iron. Massive stars over about eight times the sun’s mass that also explode as supernovae at the end of their lives produce many of the elements from carbon through to iron, including most of the oxygen and calcium needed for life.
- “Apart from hydrogen, there is no single element that can be formed only by one type of star,” explained Kobayashi. “Half of carbon is produced from dying low-mass stars, but the other half comes from supernovae. And half the iron comes from normal supernovae of massive stars, but the other half needs another form, known as Type Ia supernovae. These are produced in binary systems of low mass stars.”
- Pairs of massive stars bound by gravity, in contrast, can transform into neutron stars. When these smash into each other, the impact produces some of the heaviest elements found in nature, including gold.
- A Surprise - Supernovae with Strong Magnetic Fields:
- “Even the most optimistic estimates of neutron star collision frequency simply can’t account for the sheer abundance of these elements in the universe,” said Karakas. “This was a surprise. It looks like spinning supernovae with strong magnetic fields are the real source of most of these elements.”
- “New discoveries are to be expected from nuclear facilities around the world, including Europe, the U.S. and Japan, currently targeting rare nuclei associated with neutron star mergers,” said co-author Dr. Maria Lugaro, who holds positions at Hungary’s Konkoly Observatory and Australia’s Monash University. “The properties of these nuclei are unknown, but they heavily control the production of the heavy element abundances. The astrophysical problem of the missing gold may indeed be solved by a nuclear physics experiment.”
- The researchers concede that future research might find that neutron star collisions are more frequent than the evidence so far suggests, in which case their contribution to the elements that make up everything from mobile phone screens to the fuel for nuclear reactors might be revised upward again.
- The Daily Galaxy, Sam Cabot, via Monash University and Eurekalert and ASTRO3D
- Image credit top of page: Neutron star merger NSF/LIGO/Sonoma State University/A. Simonnet.
3. The Daily Galaxy誌の記事: 偉大な発見経路:
- LIGO: Laser Interferometer Gavitational-Wave Observatory, 米国NSFのレーザー干渉計重力波観測所 (1992), 2015/9に重力波を検出 (GW150914)
- Virgo: 伊にある重力波検出のための大型マイケルソン干渉計; 干渉検出器には指向性がないので、LIGOと共同解析して共同発表する。
- stun: 茫然とする
- globular cluster: 球状星団
- Type Ia supernova: Ia型超新星。白色矮星の激しい爆発の結果生じる。白色矮星の質量が均一なので、標準光源として利用される。
- 生物学者は、太陽光こそが金であると言うが、天文学者にとっては、宇宙に存在している金の量の探査を続けている。
- これまでの仮説では、貴金属のほとんどは中性子星の衝突によって作られたというものだった。2017/8/17に、2つの中性子星の衝突によって重力波が初めて100秒間、LIGOとVirgo (のレーザー干渉計)で観測されたことで、世界の天文学者を驚かせた。
- その事件は、GFW170817と呼ばれ、空間を伝わってきた重力波とγ線、X線、可視光、電磁波の電波が、衛星軌道上および地上の望遠鏡で観測された。
- 消えた金のミステリー:
- 銀河進化の新たな分析によれば、星の衝突は、以前想定されていた程度の元素量を生成しないとのことである。またこの研究では、現在のモデルでは宇宙における金の存在量を説明できず、天文学上のミステリーとなっているという。新たに周期律表の炭素からウランまでの元素の生成は星起源であることは示せるが、金については疑問が残るという。
- 宇宙における全ての水素 (地球上の水素を含む分子を含めて)は、ビッグバンで生成され、また大量のヘリウムとリチウムも生成されたが、その他の元素はそれほどでもなかった。その他の元素は星の内部での核融合反応で作られた。どの元素が生成されるかは、正確には星の質量によって決定され、星の最後の爆発によって、太陽風のような形で放出される。
- 圧力鍋のような星々:
- "恒星は、新たな元素を生成する圧力鍋と考えられる"と、共著者であるAmanda Karakas (豪州のASTRO 3-D准教授)は説明する。"これらの元素を生成する反応で、恒星が何十億年も輝き続けるエネルギーを得ることができる。恒星が年をとると、内部の温度が上昇するので、ますます重たい元素を生成するようになる。"
- 宇宙における闇: 目覚ましいブラックホールと中性子星の合体:
- 重力波の研究者達は、球状星団や何十万という密集した星、何百というブラックホールの集結した黒い中心部は、宇宙の至る所に発見されている。それらが重力波を生み出しているのではと思われている。
- 未発見の恒星進化プロセス:
- 全ての元素の内、半数はトリウムやウランのように鉄より重たいので、(恒星爆発の後にできる超過密な)中性子星の衝突で生成されると考えられてきた。中性子星の衝突の理論はされていたが、2017年にその衝突が確認さえた。今回の共著者であるKarakas、小林千晶、Maria Lugaroは、中性子星の役割は過大評価されており、より重たい元素の生成プロセスを別途考える必要があると指摘する。
- "中性子星の合体では、初期宇宙では重たい元素は十分には生成される140億年後でもまだ生成されない。それまでは古い恒星はそれほど存在せず、今日の重たい元素の存在比率を説明できるような十分な衝突があったとは考えられない"という。
- 研究者達が言うには、重たい元素は、全く別の恒星の現象、つまり異常な超新星が高速回転で衝突して、強力な電磁場を生成するような現象が考えられる。この発見は、Astrophysical Journal誌に公表された。この研究は、炭素からウランまでの全ての天然の元素についての恒星起源説を最初から計算し直すというものである。
- 最新モデル:
- 研究者達曰く、宇宙の進化についての現在の通説を実質的に変更する新たなモデルが必要である、と。"例えば、全ての元素が同時に生成されるモデルが必要となるが、それでも銀の生成は説明がつくは、金の生成はできない"と、英国Hertfordshire大学の小林は言う。
- "観測結果と照合すると、銀は過剰に生成され、金は過小にしか生成されない。これは、恒星爆発や核融合反応について新たな理論が必要であることを意味する。" その研究によって、今までの恒星質量、年齢、元素生成の順番を計算し直さなければならない。例えば、太陽質量の約8 倍より軽い恒星は、炭素、窒素、フッ素と同様に鉄より重たい半数の元素も生成するということになる。太陽質量の8倍より重たい恒星は、その終焉で超新星爆発を起こし、生命に必要な酸素やカルシウムを含めて、炭素から鉄まで元素を生成する、というもの。
- "水素は別として、どの元素も、一つの恒星のタイプだけで生成されるのではない"と小林は言う。"炭素の半数は、軽い質量の恒星の死によって生成されるが、残り半数の炭素は、超新星から生成される。鉄の半数は、通常の重たい恒星が超新星となって生成されるが、残り半数はType Ia型超新星から生成される必要がある。これらは低質量の連星系によって生成される。"
- 一方、重たい恒星の連星系では、中性子星に変化する。これらが衝突すると、自然界における金を含む重たい元素の一部を生成する。
- 強力な磁場を伴う驚くべき超新星:
- "中性子星が衝突する頻度についての楽観的な予測でも、これら重たい元素の宇宙での存在比を説明できない"とKarakasはいう。"驚くべきことに、強力な磁場を持った回転する超新星が、これらの元素の生成に関わっていると思われる。"
- "新たな発見は、欧米日の核融合施設が、中性子星の衝突合体に焦点を当てた研究に期待している"と共著者のDr. Maria Lugaro (Hungary Konkoly ObservatoryとAustralia's Monash University)が言う。"これらの核融合の特性は不明だが、重たい元素の生成に関わっている。消えた金の量についての天文物理学上の問題も、核融合物理実験で解決されると思う。"
- 研究者達は、中性子星の衝突は、従来確認されているよりもっと頻繁に起こるのではと認めており、その場合、携帯電話のスクリーンから原子炉の燃料まで利用されている元素の生成について修正がなされることになろうと言う。
>Top 4. Notimérica: Ciencia-Tecnología, Madrid, 15 Sep., 2020:
- Una tabla periódica de nueva apariencia por las estrellas de neutrones:
- Las colisiones de estrellas de neutrones no crean la cantidad de elementos químicos que se suponía hasta ahora, según ha descubierto un nuevo análisis de la evolución de las galaxias.
- También revela que los modelos actuales no pueden explicar la cantidad de oro en el cosmos, lo que despierta un misterio astronómico.
- El trabajo, publicado en Astrophysical Journal, ha producido una tabla periódica de nueva apariencia, que muestra los orígenes estelares de elementos naturales desde el carbono hasta el uranio.
- Todo el hidrógeno del Universo, incluidas todas sus moléculas en la Tierra, fue creado por el Big Bang, que también produjo mucho helio y litio, pero no mucho más.
- El resto de los elementos naturales están hechos a partir de diferentes procesos nucleares que ocurren dentro de las estrellas. La masa gobierna exactamente qué elementos se forjan, pero todos se liberan en las galaxias en los momentos finales de cada estrella, explosivamente en el caso de las realmente grandes, o como salidas densas, similares al viento solar, para las de la misma clase que el Sol.
- "Podemos pensar en las estrellas como ollas a presión gigantes donde se crean nuevos elementos", explica el coautor, profesor asociado Karakas, del Centro de Excelencia ARC de Australia para la Astrofísica del Cielo en 3 Dimensiones (ASTRO 3D).
- "Las reacciones que producen estos elementos también proporcionan la energía que mantiene a las estrellas brillando durante miles de millones de años. A medida que las estrellas envejecen, producen elementos cada vez más pesados a medida que su interior se calienta", añade.
- Se pensaba que la mitad de todos los elementos que son más pesados ??que el hierro, como el torio y el uranio, se formaron cuando las estrellas de neutrones, los restos superdensos de soles quemados, chocaron entre sí. Las colisiones de estrellas de neutrones, teorizadas durante mucho tiempo, no se confirmaron hasta 2017.
- Ahora, sin embargo, un nuevo análisis de Karakas y sus compañeros astrónomos Chiaki Kobayashi y Maria Lugaro revela que el papel de las estrellas de neutrones puede haber sido considerablemente sobrestimado, y que otro proceso estelar en conjunto es responsable de producir la mayoría de los elementos pesados.
- "Las fusiones de estrellas de neutrones no produjeron suficientes elementos pesados en la vida temprana del Universo, y todavía no lo hacen ahora, 14.000 millones de años después --añade Karakas--. El Universo no los hizo lo suficientemente rápidos para dar cuenta de su presencia en estrellas muy antiguas y, en general, simplemente no hay suficientes colisiones para explicar la abundancia de estos elementos en la actualidad".
- En cambio, los investigadores encontraron que los elementos pesados ?debían ser creados por un tipo completamente diferente de fenómeno estelar: supernovas inusuales que colapsan mientras giran muy rápido y generan fuertes campos magnéticos.
- El hallazgo es uno de los varios que surgen de su investigación, que acaba de ser publicada en el 'Astrophysical Journal'. En este estudio es la primera vez que los orígenes estelares de todos los elementos naturales, desde el carbono hasta el uranio, se han calculado a partir de los primeros principios.
- El nuevo modelo, dicen los investigadores, cambiará sustancialmente el modelo actualmente aceptado de cómo evolucionó el universo. "Por ejemplo, construimos este nuevo modelo para explicar todos los elementos a la vez y encontramos suficiente plata pero no suficiente oro", señala el coautor, profesor asociado Kobayashi, de la Universidad de Hertfordshire, en el Reino Unido.
- "La plata se produce en exceso, pero el oro se produce menos en el modelo en comparación con las observaciones. Esto significa que es posible que debamos identificar un nuevo tipo de explosión estelar o reacción nuclear", añade.
- El estudio refina estudios previos que calculan los roles relativos de la masa, la edad y la disposición de las estrellas en la producción de elementos. Por ejemplo, los investigadores establecieron que las estrellas más pequeñas que unas ocho veces la masa del Sol producen carbono, nitrógeno y flúor, así como la mitad de todos los elementos más pesados que el hierro.
- Las estrellas masivas de aproximadamente ocho veces la masa del Sol que también explotan como supernovas al final de sus vidas, producen muchos de los elementos desde el carbono hasta el hierro, incluida la mayor parte del oxígeno y el calcio necesarios para la vida.
- "Aparte del hidrógeno, no existe un elemento único que pueda estar formado por un solo tipo de estrella --explica Kobayashi--. La mitad del carbono se produce a partir de estrellas moribundas de baja masa, pero la otra mitad proviene de supernovas. Y la mitad del hierro proviene de supernovas normales de estrellas masivas, pero la otra mitad necesita otra forma, conocida como supernovas de Tipo Ia. Estas se producen en sistemas binarios de estrellas de baja masa".
- Por el contrario, pares de estrellas masivas unidas por la gravedad pueden transformarse en estrellas de neutrones. Cuando estos chocan entre sí, el impacto produce algunos de los elementos más pesados que se encuentran en la naturaleza, incluido el oro.
- En el nuevo modelo, sin embargo, los números simplemente no cuadran. "Incluso las estimaciones más optimistas de la frecuencia de colisión de estrellas de neutrones simplemente no pueden explicar la gran abundancia de estos elementos en el Universo --asegura Karakas--. Esto fue una sorpresa. Parece que las supernovas giratorias con fuertes campos magnéticos son la fuente real de la mayoría de estos elementos".
- La coautora, la doctora Maria Lugaro, que ocupa puestos en el Observatorio Konkoly de Hungría y en la Universidad Monash de Australia, cree que el misterio del oro perdido podría resolverse muy pronto. "Se esperan nuevos descubrimientos de las instalaciones nucleares de todo el mundo, incluidos Europa, Estados Unidos y Japón, que actualmente apuntan a núcleos raros asociados con fusiones de estrellas de neutrones", añade.
- "Se desconocen las propiedades de estos núcleos, pero controlan en gran medida la producción de la abundancia de elementos pesados --prosigue--. El problema astrofísico del oro perdido puede ser resuelto por un experimento de física nuclear".
- Los investigadores admiten que la investigación futura podría encontrar que las colisiones de estrellas de neutrones son más frecuentes de lo que sugiere la evidencia hasta ahora, en cuyo caso su contribución a los elementos que componen todo, desde las pantallas de los teléfonos móviles hasta el combustible de los reactores nucleares, podría revisarse nuevamente al alza.
- Leer más: https://www.notimerica.com/ciencia-tecnologia/noticia-tabla-periodica-nueva-apariencia-estrellas-neutrones-20200915174256.html
(c) 2020 Europa Press. Está expresamente prohibida la redistribución y la redifusión de este contenido sin su previo y expreso consentimiento.
4. Notimérica: Science-Technology:
- A new-looking periodic table for neutron stars:
- The collisions of neutron stars don't create the amount of chemical elements previously assumed, a new analysis of the galaxy evolution has found.
- It also reveals that current models cannot explain the amount of gold in the cosmos, which raised an astronomical mystery.
- The work, published in the Astrophysical Journal, has produced a new-looking periodic table, showing the stellar origins of natural elements from carbon to uranium.
- All hydrogen in the universe, including all its molecules on earth, was created by the Big Bang, which also produced a lot of helium and lithium, but not so much else.
- Rest of the natural elements are made from different nuclear processes that occur within stars. Mass governs exactly which elements are forged, but they are all released into galaxies in each star's final moments, explosively in the case of really large ones, or as dense outlets, similar to the solar wind, for those of the same class as sun.
- "We can think of stars as giant pressure cookers where new elements are created," explains co-author, Associate Professor Karakas, from Australia's ARC Center of Excellence for Astrophysics of the Sky in 3 Dimensions (ASTRO 3D).
- "The reactions where these elements are produced also provide energy that keeps stars shining for billions of years. As stars age, they produce heavier and heavier elements as their interior warms up," she adds.
- Half of all elements that are heavier than iron, such as thorium and uranium, were thought to be formed when neutron stars, the super-dense remnants of burning suns, collided with each other. Neutron star collisions, long theorized, were not confirmed until 2017.
- Now, however, a new analysis by Karakas and fellow astronomers Chiaki Kobayashi and Maria Lugaro reveals that the role of neutron stars may have been considerably overestimated, and that another stellar process as a whole is responsible for producing most of the heavy elements.
- "Neutron star mergers did not produce enough heavy elements in the early life of the Universe, and they still do not now, 14 billion years later," Karakas adds. "The Universe did not make them fast enough to account for their presence in very old stars and, in general, there are simply not enough collisions to explain the abundance of these elements today. "
- Instead, the researchers found that the heavy elements - must be created by an entirely different type of stellar phenomenon: unusual supernovae that collapse while spinning very fast and generate strong magnetic fields.
- The finding is one of several to emerge from their research, which has just been published in the Astrophysical Journal. This study marks the first time that the stellar origins of all natural elements, from carbon to uranium, have been calculated from first principles.
- The new model, the researchers say, will substantially change the currently accepted model of how the universe evolved. "For example, we built this new model to explain all the elements at once and we found enough silver but not enough gold," says co-author, Associate Professor Kobayashi, from the University of Hertfordshire in the UK.
- "Silver is produced in excess, but gold is produced less in the model compared to observations. This means that we may need to identify a new type of stellar explosion or nuclear reaction," she adds.
- The study refines previous studies that calculate the relative roles of the mass, age and arrangement of stars in the production of elements. For example, the researchers established that stars smaller than about eight times the mass of the Sun produce carbon, nitrogen, and fluorine, as well as half of all elements heavier than iron.
- Massive stars roughly eight times the mass of the Sun that also explode as supernovae at the end of their lives produce many of the elements from carbon to iron, including most of the oxygen and calcium needed for life.
- "Aside from hydrogen, there is no single element that can be made up of just one type of star," Kobayashi explains. "Half of the carbon is produced from low-mass dying stars, but the other half comes from supernovae. And half of the iron comes from normal supernovae in massive stars, but the other half needs another form, known as Type Ia supernovae. These occur in low-mass binary star systems. "
- In contrast, pairs of massive stars held together by gravity can transform into neutron stars. When these collide, the impact produces some of the heaviest elements found in nature, including gold.
- On the new model, however, the numbers just don't add up. "Even the most optimistic estimates of the collision frequency of neutron stars simply cannot explain the great abundance of these elements in the Universe - Karakas says -. This was a surprise. It seems that rotating supernovae with strong magnetic fields are the actual source of most of these elements. "
- Co-author Dr Maria Lugaro, who holds positions at Hungary's Konkoly Observatory and Australia's Monash University, believes the mystery of the lost gold could be solved very soon. "New discoveries are expected from nuclear facilities around the world, including Europe, the United States and Japan, which currently point to rare nuclei associated with neutron star mergers," she adds.
- "The properties of these nuclei are unknown, but they control to a great extent the production of the abundance of heavy elements - she continues -. The astrophysical problem of lost gold can be solved by a nuclear physics experiment."
- The researchers admit that future research could find that neutron star collisions are more frequent than the evidence so far suggests, in which case their contribution to the elements that make up everything from cell phone screens to fuel of nuclear reactors, could be revised up again.
4. Notimérica: Ciencia-Tecnologia誌の記事:
- 中性子星による新たな周期律表は:
- これまで想定されてきた量の化学元素は中性子星の衝突では生成されない、との新たな銀河系の進化による分析結果が示された
- また今のモデルでは天文学上の謎とされてきた宇宙での金の存在量が説明できない。
- 米国Astrophysical Jounal誌によれば、炭素からウランまでの天然元素の起源が示された新たな周期律表が発表された。
- 宇宙にある全ての水素 (地球上にある分子を構成するものを含め)、及び多くのヘリウムとリチウムは、ビッグバンで生成されたが、その他はそれほど多くはない。
- その他の天然の元素は、恒星内部で起こる異なった核融合プロセスで生成される。質量がどの元素が生成されるのかが決定的に決まり、それらは全て、重い恒星の場合は爆発的に、太陽程度の場合は、太陽風のような濃密な流れとなって、恒星の最後の段階で宇宙空間に放出される。
- "恒星は、新たな元素を生成する巨大な圧力鍋と考えることができる"と共著者であるKarakas准教授 (豪州 ASTRO 3D)は言う。
- これらの元素が生成される反応は、恒星が何十億年に亘って輝き続けるエネルギーを供給する。恒星が年老いると、内部の温度上昇に伴って、ますます重たい元素を生成する。
- 半数の元素は、トリウムやウランなど鉄より重たい元素で、それらは中性子星 (爆発した恒星の残滓で超高密度; 1㎤当り5億トン) 同士が衝突する。この中性子星衝突が原因であると長い間理論づけられてきましたが、2017年にはそうでないことが示された。
- しかし、Karakas, Chiaki Kobayashi, Maria Lugaroの研究者チームは、中性子星の役割はかなり過大評価されていることを示し、その他の恒星のプロセスがほとんどの重たい元素生成に関わっていることを明らかにした。
- "中性子星は、初期宇宙において十分な重たい元素を生成しなかったし、それは140億年後の現在もそうある"と、Karakasはさらに言う。"宇宙は、非常に古い星の内部で重たい元素を生成するには時間が十分とは言えなかったし、さらに今日の重たい元素の存在比を説明できるほどの衝突があったのは言えない。"
- そうではなくて研究者達が見つけたのは、重たい元素は全く異なるタイプの恒星現象、即ち、超新星が非常に早く回転して強力が磁場を発生している間に衝突するという特殊な現象だったに違いない。
- 彼等の研究が出てきたこの発見について、最近Astgrophysical Journal誌に掲載された。この研究は、炭素からウランまでの全ての天然の元素について恒星起源を原理に基づく計算に基づいている。
- 研究者達が言うには、この新たなモデルは、宇宙の進化についての今までの通説をかなり変えるものになろう。"例えば、この新モデルは全ての元素生成を一度に説明できるが、なお銀については多すぎ、また金については少なすぎるという面はある"と共著者のKobayashi准教授・英国University of Hertfordshire)は言う。
- "観測データと比べると銀の生成は過剰だが、金の生成は過小となる。これはさらに恒星爆発あるいは核融合について新たなタイプを見つけなければならないことを意味する。"と、彼女は付け加える。
- この研究は、それまでの研究結果である元素生成における星の質量、年齢、星の配列を修正するものである。例えば、太陽質量より8倍未満の場合は炭素、窒素、フッ素などと鉄より重たい半数の元素も生成することを示した。
- 太陽質量より8倍も重たい恒星は、その寿命の最後に超新星爆発を起こし、生命に必要な酸素やカルシウムを含め、炭素から鉄までの多くの元素を生成する。
- "水素は別だが、その他の元素はどれも一つのタイプの恒星から生成される訳ではない"とKobayashiは説明する。"炭素の半分は、質量の軽い白色矮星から、残りの半数は超新星から生成される。また鉄の半数は通常の超新星爆発起源だが、残りの半数は、Ia型超新星という別の型式から生成される。この型は低質量の連星系から生じる。"
- 対照的に、重質量の恒星同士は重力によって中性子星に変化する。これらが衝突すると、その衝撃で、金を含む自然界で最も重たい元素の一部が生成される。
- 新モデルでは、それでも数字が合わなかった。"中性子星の衝突頻度を最大限楽観的に見積もっても、宇宙におけるこれらの元素の存在比を説明できない"とKarakasは言う。驚くべきことに、強力な磁場を持った回転している超新星は、これら多くの元素の実際の起源になっているようだ。"
- 共著者であるDr. Maria Lugaro (ハンガリーのKonkoly Observatory及び豪州のMonash University)は、失われた金の謎についてはまもなく解明されると思う。"欧州、米国、日本など世界の核融合施設は、現在、中性子星の合体に関連した稀な核に焦点を当たっており、近々新たな発見が期待できる"とのこと。
- "これらの核の特質はまだ不明だが、重たい元素の存在比を示すような生成メカニズムをコントロールしており、失った金という天文学上の問題につていも、核物理学実験によって解決され得るだろう”とのこと。
- 研究者達が、将来の研究によって中性子星衝突が、従来言われてきたよりもっと頻度が高くて、 (携帯電話のスクリーンから原子炉の燃料に至る.)元素の生成過程に寄与できているかは、今後修正が加えられることになろう、と。
>Top 5. VICE: Becky Ferreira, 18 Sept. 2020:
- Scienctists hunt for the source of the universe's 'Missing Gold':
Heavy elements like gold are created by the deaths, explosions, and collisions of stars. A new study breaks down these diverse origins.
- We are made of “star stuff,” in the words of astronomer Carl Sagan, which is a poetic reminder that life on Earth is interlinked with cosmic processes that date back billions of years.
- But exactly which stars make what stuff is still a matter of some debate. While we know that heavy elements such as gold, silver, and iron are forged by the deaths of stars, or in cataclysmic stellar collisions, it’s not clear what proportion of elements originate from each source.
- That’s why a team of astrophysicists developed an updated Periodic Table that includes the stellar origins of elements ranging from carbon through uranium. The results reveal a mysterious gap in our knowledge about the genesis of gold in the cosmos, among many other insights published on Tuesday in The Astrophysical Journal.
- “We were interested in testing theoretical predictions for various ‘sites’ of element production,” said co-author Amanda Karakas, an astrophysicist at Monash University in Australia, in an email. “By ‘sites’ I mean types of stars, as different types of stars can make elements depending on their mass.”
- Karakas, along with authors Chiaki Kobayashi of the University of Hertfordshire in the U.K. and Maria Lugaro of Konkoly Observatory in Hungary, also emphasized that these elemental lineages are key to understanding the intricate evolution of the galaxies as a whole.
- “Stars in the present-day galaxy are fossils that retain the information on the properties of stars from the past,” the team said in the study. “This approach is called Galactic archaeology and can be applied not only to our Milky Way Galaxy but also to other galaxies.”
- Scientists are able to estimate the abundance and origin of elements through a variety of techniques. Details about the chemical composition of a star can be directly observed in its light spectrum, and stellar evolution models predict which elements should be created by stars depending on their mass, age, and location.
- The Big Bang, the event that kicked off the universe, is the main source of the lightest elements: hydrogen, helium, and lithium. Stars, meanwhile, forge complex heavy elements, including those that are essential for life, such as carbon, oxygen, and nitrogen, or flashy metals like gold, silver, and iron.
- The origins of nearly all elements can be traced to several sources, but the new study constrains the contribution of each type of stellar death, explosion, or crash. Modeling such a complex problem requires estimates of the abundance of various star types across time and space, as well as the frequency of the powerful cosmic processes that produce them.
- “We wanted to know the origin of elements exactly, ie. how much fraction of each element is produced from what kind of stars,” said Kobayashi in an email.
- Previous studies have suggested that collisions between neutron stars, the dead husks of some massive stars, could be a major source of heavy elements, including gold. But the new study challenges the assumption that neutron star mergers play a dominant role in gilding the universe.
- “We were interested in heavy elements because neutron star mergers were recently hypothesised to produce half of the elements heavier than iron by the ‘rapid’ neutron capture process,” Karakas said.
- “We wanted to know if that was the case, using state-of-the art neutron star merger theoretical calculations,” she continued. “We found that neutron star mergers do not occur quickly enough to account for the observed heavy element composition of very ancient stars nor can it produce enough to account for the amounts of heavy elements observed in our Solar System.”
- The researchers suggest that a special type of rapidly spinning supernova, with strong magnetic fields, might explain this “mystery of the missing gold,” as it was described in a release.
- “We found that a rare class of rapidly rotating, highly magnetic supernovae makes most of the heavy elements via the rapid process,” Karakas said. “However, we still don't know how many massive stars end their lives this way, nor many of the details of the explosions.”
- “We are already seeing considerable progress with 3D models and I suspect in the next five years we will have a better handle on these questions,” she added.
- To shed more light on elemental origins, scientists will need to crack the weird nuclear physics that govern the rapid neutron capture process that creates gold and other heavy elements. Next-generation nuclear facilities may be able to constrain the dynamics of this particular process.
- In addition to nuclear experiments in laboratories on Earth, Kobayashi noted that “the observation of ancient ‘metal-poor’ stars that were born just after the Big Bang, much earlier than the Solar System, is very useful.”
- “In stellar spectra, it is very difficult to measure very heavy elements in general, and is extremely hard to measure gold,” she said. “Gold has been observed only for a handful metal-poor stars and in the Sun so far, but more measurements will be obtained in the near future (with the Hubble Space Telescope).”
- It’s also possible that the team underestimated the number of neutron star collisions, given that astronomers have only just begun to directly observe these wild encounters within the past few years using new gravitational wave detectors called LIGO and Virgo.
- “Our predictions regarding the amount of heavy elements associated with neutron star mergers depends on how quickly two neutron stars can merger, and also the rates of these events in the Galaxy,” Karakas said. “Hopefully, neutron star merger rates will be much better constrained in the next 10 years by results from the LIGO/Virgo collaborations, which look for the gravitational wave signatures of such events.”
- The breakdown of elements that living stars will create, and eventually sow into the universe, are helpfully visualized in the above Periodic Table. Stars that are over eight times as massive as the Sun are destined to explode into pyrotechnic supernovae. This process is the main source of certain elements, such as neon, silver, and iridium.
- Smaller stars, like the Sun, don’t undergo a supernova event. As they are dying, these stars shed their outer gassy layers into their surroundings, a dynamic that enriches the next generation of stars with new elements. This process is a major source of nitrogen, lead, and strontium.
- The burned-out corpses of stars like the Sun, known as white dwarfs, sometimes explode in a special type of supernova that supplies much of the universe’s manganese, iron, and nickel.
- The phrase “star stuff” encapsulates all of these intricacies in a lovely and succinct way. But behind those simple words lies a mountain of fascinating and ever-evolving research into the mind-boggling variety of elemental origin stories out there in the stellar wilds.
- Update: This article has been updated with comments from Dr. Karakas and Dr. Kobayashi.
5. VICE: Becky Ferreira:
- star stuff: 星の材料
- intricacy: complexity
- encapsulate: enclose in a capsule
- succint: briefly and clearly
- boggle: be astonished
- wild: natural state or uncultivated
- 科学者は、宇宙での’消えた金’の原因を探索中。
- 金のような重金属は、恒星の死、爆発、衝突によって生成される。これらの様々な起源について新たな研究結果が出た。
- 天文学者Carl Saganの言葉によれば、我々は"星の子である"と、地球上の生命は、数十億年前に遡る宇宙のプロセスと結びついていると詩的に表現されている。
- しかし、正確には、どの恒星のどの材料がそのようになるのかについては議論の余地がある。我々が今までの認識では、金、銀、鉄などの重金属は、恒星の死によるか、あるいは恒星の劇的な衝突によって生成されるのか、その割合はどうなのかについて明確ではなかった。
- 今回、宇宙物理学者のチームは、炭素からウランに至る周期律表の元素の起源を明らかにした。その結果は、9/15にThe Astrophysical Journal誌に公表され、宇宙における金の生成についての我々の知識の謎を埋めるものであった。
- ”我々は、元素の生成についてさまざまな場所に対する理論的な予測に関心がある”と、共著者であるAmanda Karakas (豪州Monash University)はemailで回答した。"その場所とは、恒星の質量の違いによって様々な種類の生成の場という意味です。"
- Karakasが言うには、Chiaki Kobayashi (英国University of Hertfordshire、およびMaria Lugaro (ハンガリーKonkoly Observatory)との共著者で、元素間の関連性が、全体として複雑な銀河の進化を理解する上で鍵となる、と。
- "今日の銀河における恒星は、過去からの恒星の特質に間s塗る情報を保持している化石であり、このやり方は、銀河考古学と呼ばれ、我々の銀河系のみならず他の銀河系にも適用可能である。"と語った。
- 科学者は、様々な技術を使って、元素の存在量と起源と推定することができる。恒星の化学的構成についえはその光のスペクトルから直接的観測でき、恒星の進化モデルによってどの元素が、恒星の質量、年齢、位置関係によって生成されるのかはわかる。
- 宇宙が始まったビッグバンによって、軽い元素、即ち、水素、ヘリウム、リチウムが生成された。一方で、恒星は複雑な重金属元素、生命に必須のものを含む、炭素、酸素、窒素、や金、銀、鉄などが生成された。
- ほぼ全ての元素の起源については、いくつかの成因を追えるが、今回の新たな研究は、恒星の死、爆発、あるいは崩壊といった各タイプがそれぞれの元素の生成にどう貢献したのか特定した。このような複雑な問題をモデリングするには、各種恒星の時間・空間別の型や、元素生成に関わる強力な宇宙プロセスの頻度などを必要とする。
- "我々は元素の起源を正確に知りたかった。即ち、それぞれの元素が恒星のどの種類によって生成されたのかということである"と、Kobayashiはemailで回答した。
- 以前の研究では、中性子星の衝突、一部の巨大な恒星の爆発後の殻が、金などの重元素の主な起源だった。しかし新たな研究結果では、中性子星の合体が、元素生成の主な原因を推測される。
- "我々が、重元素の生成に着目したのは、中性子星の合体によって、'急速な'中性子捕獲プロセス (r-process)によって、鉄より重たい元素の半数の生成に関与しているという仮説によるものである"と、Karakasは言う。
- "我々は、最新の中性子星の合体理論の計算結果を使って検証した。その結果、中性子星の合体は、非常に古い恒星では重たい元素はそれほど説明できず、また我々の太陽系で観察される程の量は生成されないことがわかった。"
- 研究者達が言うには、強力な磁場を持ち、急速に回転している超新星が、この"失われた金"の説明ができるのではと論文で述べている。
- "我々としては、r-processよりは、高速に回転して強力磁場を持つ超新星がほとんどの重金属を生成したと考える"とKarakasは言う。"しかし、どれ程多くの重たい恒星が最後の時を迎えるのか、その爆発の詳細をまだよくわかっていない。"
- "我々はすでに3Dモデルの進歩にかなりわかるようになったが、今後5年間以内に、さらに疑問が解けるようになると思われる"と彼女は加えた。
- 元素の起源解明にさらに光を当たるために、科学者達は、奇妙な核物理学を使って、金その他の重金属を生成する高速中性子捕獲プロセス解明する必要がある。次世代の核融合施設はこの特殊なプロセスの力学を切り開くことができるかも知れない。
- 地上の核融合実験装置に加えて、ビッグバン直後に生成された金属量の少ない、太陽系よりも遥かに古い恒星を観察することは非常に有効だと思うとKobayashiは指摘する。
- "恒星のスペクトルでは、非常に重たい元素、特に金を測定することは、一般的には非常に難しい"と彼女は語る。"金は、今まではほんのわずかな金属の少ない恒星および太陽で観測されてきたが、今後はHubble Space Telescopeなどによって、もっと測定が増えると思う。"
- ここ数年の間にLIGOやVirgoといった新たな重力波を使ってさらに激しい現象を直接観測できるようになったことを踏まえると、研究チームは、中性子星衝突の数を過小評価してきたという可能性もあり得る。
- "中性子星合体による重金属の量については、2つの中性子星がどのように早く合体するのか、またこの合体という現象がどの程度に頻度で起こるのか次第となる"と、Karakas は言う。"中性子星の合体する確率は、今後10年間に、この現象で重力波を検出しようというLIGOとVirgoの共同研究の結果で、もっとよく解明されると期待している。"
- 恒星が生きている間に生成して宇宙に拡散する元素を分類することは、前述の周期律表で明示的に説明できる。太陽質量の8倍を超える重たい恒星は、華々しい超新星爆発を起こすことが運命づけられている。このプロセスは、ネオン、銀、イリジウムなど一部の元素の主要な原因となっている。一方、太陽のように軽い星は、超新星爆発をおこなし。それらは、死滅する際に、外殻のガス層を周囲に放出し、次世代の星が誕生する際の新たな元素を追加する。このプロセスによる元素生成は、窒素、鉛、ストロンチウムなどである。
- 太陽のサイズの星が燃え尽きると、白色矮星となり、それは時々特殊な型の超新星爆発を起こし(Ia型超新星)、宇宙におけるマンガン、鉄、ニッケルを生成する。
- "星の材料"という言葉には、これらのような素敵で複雑な意味が込められている。この単純な言葉は、星の姿が解明されるに従って元素の起源という心躍る物語を生み出していく。
- 本論文は、Dr. KarakasとDr. Kobayashiのコメントによって更新された。
>Top 6. Cf: Type Ia Supernova: (SNIa) (by Wikipedia)
- Single degenerate progenitors:
- One model for the formation of this Type Ia Supernova is a close binary star system; the primary star possess more mass than the secondary. Being greater in mass, the primary star evolves onto the asymptotic giant branch, where the star's envelope expands considerably.
- If the two stars share a common envelope then the system can lose significant amounts of of mass, reducing the angular momentum, orbital radius and period.
- >Top After the primary has degenerated into a white dwarf, the secondary star later evolves into a red giant and the stage is set for mass accretion onto the primary. During this final shared-envelope phase, the two stars spiral in closer together as angular momentum is lost. The resulting orbit can have a period as brief as a few hours. If the accretion continues long enough, the white dwarf may eventually approach the Chandrasekhar limit. (about 1.4 M⦿ $\;=2.765 \times 10^30$kg; white dwarfs resist gravitational collapse primarily through electron degeneracy pressure.)
- The white dwarf companion could also accrete matter from other types of companions, including a sub-giant or even a main sequence star. The actual evolutionary process during this accretion stage remains uncertain. It has been estimated that single degenerate progenitors account for no more than 20% of all Type Ia supernovae.
- >Top Double degenerate progenitors:
- A second possible mechanism is the merger of two white dwarfs; which is called a super-Chandrasekhar mass white dwarf. In such a case, the total mass would not be constrained by the Chandrasekhar limit.
- >Top Collision of solitary stars within the Milky Way occur only once every $10^7$ to $10^13$ years; far less frequently than the appearance of novae. Collisions occur with greater frequency in the dense core regions of globular clusters*. A likely scenario is a collision with a binary star system, or between two binary systems containing white dwarfs. This collision can leave behind a close binary system of two white dwarfs. Their orbit decays and they merge through their shared envelope. It is implied that a double white dwarf merger every 100 years in the Milky Way; this rare matches the number of Type Ia supernovae detected in our neighborhood.
- Double degenerate scenarios raise questions about the applicability of Type Ia supernovae as standard candles, since total mass of the two merging white dwarfs varies significantly, meaning luminosity also varies.
- Light curve:
- Type Ia supernovae have a characteristic light curve, their graph of luminosity as a function time after the explosion. Near the time of maximal luminosity, the spectrum contains lines of intermediate mass elements from O to Ca; there are the main constituents of the outer layers of the star. Months after the explosion, when the outer layers have expanded to the point of transparency, the spectrum is dominated by light emitted by material near the core of the star, heavy elements synthesized during the explosion; most prominently isotopes close to the mass of Fe. The radioactive decay of Ni-56 to Fe-56 produced high-energy photons, which dominated the energy output of the ejecta at intermediate to late times.
- In 1998, observations of distant Type Ia supernovae indicated the unexpected result that the universe seems to undergo an accelerating expansion.
- >Top Light curve of Type Ia supernova:
- This plot of luminosity (raltive to Sun, L0) versus time shows the characteristic light curve.
- The peak is primarily due to the decay of Ni, while the later stage is powered by Co.
6. <参考>: Ia型超新星: (Wikipedia)
- binary star: 連星、双子星。2つの恒星が、両者の重心を軌道運動している天体。肉眼で見える恒星の半数以上が連星である可能性がある。明るい方を主星、暗い方を伴星と呼ぶ。連星は軌道計算から恒星の質量が求められる。
- accretion: 付着・堆積による成長
- progenitor: 先祖、先駆
- >Top Asymptotic Giant Branch (AGB): 漸近巨星分枝。小中質量星(0.8-8 M⦿)は全てその生涯の後半にこの段階に進化する。太陽より数千倍明るい赤色巨星のように見える。不活発な中心核はO/C、Heの核融合でCが形成される殻、Hの核融合でHeが形成される殻、通常の恒星の化学組成を持つ大きな外層という構造を持つ。
- *globular cluster: 球状星団; 恒星が互いの重力で球状に集まった天体。かつては母銀河の周囲を回る伴銀河であった、外側の星々が潮汐力で母銀河に飲み込まれ、密度の高い中心核のみ残って球状星団に変化した可能性。年齢が古く(ほぼ宇宙年齢)星団内の星がほぼ同時に作られた。なお、弓道星団は、銀河を囲む球対称のハロー内に分布しているので、観測で見える球状星団の分布が非対称であることから太陽は直径10万光年の銀河中心から26千年光年離れた位置にあることが判明。
- supernova remnant (SNR): 超新星残骸。外層のガスは衝撃波による断熱圧圧縮や放射性元素の崩壊熱で非常に高温(1M K以上)となり光を放射する。超新星残骸は高温状態が維持できなく成るまで数万年輝き続ける。(1054年出現のかに星雲など)
- 単一縮退形成モデル:
- Ia型超新星形成モデルの一つは、近接した連星系の場合で、主系列星と小質量の伴星から成る。主星が先に'漸近巨星分枝'に進化し、恒星の外層が肥大化する。
- 2つの恒星が外層を共有すると、系は多くの質量を失い、角モーメント、軌道半径、起動周囲を現象させる。
- 主星が白色矮星に縮退すると、伴星は赤色巨星に進化し、主星に質量転移を始める。この外層を共有する最終段階で、2つの恒星は螺旋を描きながら接近し、角モーメントは失われ、周期が数時間程度まで短くなる。物質の降着が長く続くと、白色矮星はチャンドラセカール限界に近づく。
- 複数縮退形成モデル:
- 2つの白色矮星が融合して、合計質量がチャンドラセカール限界を超える場合がある(=超チャンドラセカール質量矮星)。この場合、チャンドラセカール限界に製薬されない。
- 恒星同士の衝突は、銀河系内では$10^7 - 10^{13}$年に一度起きると考えられるが、これは新星の出現より低頻度である。しかし衝突は、球状星団等の密度の高い核の領域では、より頻繁に起きる。あり得るのは、白色矮星を含む連星系内の、または連星系同士の衝突である。この衝突の結果、2つの白色矮星からなる接近した連星系が後にできる。これらの起動は徐々に減衰し、共有する外層を通じて融合する。
- Type Ia Supernova: (Wikipedia)
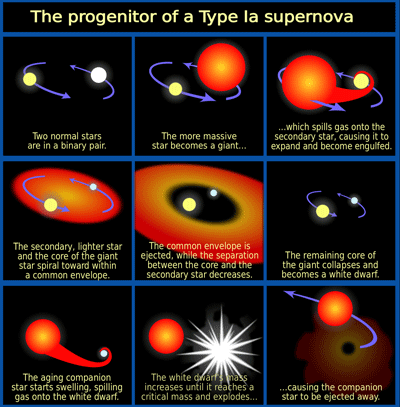
- 光度曲線:
- Ia型超新星は、特徴的な高度曲線を持つ。最高高度の近辺でのスペクトルは、OからCaまでの元素の吸収線を含む。爆発から数ヶ月経つと、スペクトルは重元素に由来するものが主となる。Ni-56は、Co-56を経てFe-56に崩壊し、その際高エネルギーの光子を生産する。
>Top 7. Cf: Distance measurement by Type Ia Supernova: (Hubblesite)
- To find distanced in space, 'standard candles which gives a certain amount of light are used. For example, if you're standing on a street evenly lined with lampposts, the second lamp will look 1/4 as bright as the first street lamp, and the third will look 1/9, and so on.
- >Top For short distances in space - within our local group of nearby galaxies - astronomers use a type of star called a Cepheid variable as a standard candle. To measure distanced to far-flung galaxies, astronomers need to find incredibly bright objects such as supernova.
- Supernovae, which occur within a galaxy about every 100 years, are among the brightest events in the sky. To determine distances, astronomers use a certain type of exploding star called a Type Ia supernova, which occur in a binary system. One of the stars in the system must be a white dwarf star, the dense, carbon remains of a star that was about the size of our Sun. The other can be a giant star or even a smaller white dwarf.
- White dwarf stars are one of the densest forms of matter, second only to neutron stars and black holes. (Just a teaspoon of matter from a white dwarf would weigh 5t.) The white dwarf will begin to pull material off its companion star, adding the matter to itself. When the white dwarf reaches 1.4 solar masses, a nuclear chain reaction occurs, causing the white dwarf to explode. The resulting light is 5 billion times brighter than the Sun.
- Because the chain reaction always happen in the same way, and the at the same mass, the brightness of these Type Ia supernovae are also always the same. The explosion point is known as the Chandrasekhar limit.
- To find the distance to the galaxy that contains the supernova, scientists just have to compare how bright they know the explosion should be with how bright the explosion appears. Using the inverse square law, they can compute the distance to the supernova and thus to the supernova's home galaxy.
7. <参考>: Ia型超新星による距離測定: (Wikipedia)
- binary star: 連星、双子星。2つの恒星が、両者の重心を軌道運動している天体。肉眼で見える恒星の半数以上が連星である可能性がある。明るい方を主星、暗い方を伴星と呼ぶ。連星は軌道計算から恒星の質量が求められる。
- accretion: 付着・堆積による成長
- elecgtron degeneracy pressure: 電子縮退圧
- 空間の距離を測るには、一定の光量の'標準ロウソク'を利用する。例えば、等間隔に並んだ街灯を眺めると、二番目の街灯の明るさは一番目の1/4になり、三番目は1/9のように暗く見える。
- 我々の銀河系の周辺など比較的近距離の場合は、ケフェウス型変光星を標準光源として使う。遥か遠方の銀河までの距離を測定するには、超新星のように異常に明るい物体を使う。
- 超新星は、銀河の中では約100年に一回起き、天空で最も明るい現象である。距離を測定するには、連星系の中で発生するIa型超新星を利用する。連星系の恒星の一つ場合は、白色矮星であって、それは高密で、Cが残っている恒星で、サイズは太陽程度。他の場合は、巨星またはより小さな白色矮星の場合もある。
- 白色矮星は、ブラックホール、中性子星に次ぐ高密度の物質である。(白色矮星は1㎤で1.1トン, 中性子星は10億トンもある) 白色矮星は、その伴星かr物質を剥ぎ取り自身に追加し始める。白色矮星が太陽質量の1.4倍に達すると、核融合反応が発生し、白色矮星は爆発し、その時に光度は、太陽の50億倍も明るくなる。
- 核融合反応は、常に一方向に、同じ質量で発生するので、Ia型超新星の明るさは一定である。この爆発点はチャンドラセカール限界と呼ばれる。
- 銀河までの距離を測定するには、そこに含まれる超新星爆発による明るさがどの程度かによって銀河の明るさの比較ができる。逆二乗の法則により、超新星までの距離、つまりそれの属する銀河までの距離を計算できるのである。
>Top 8. Physicsworld (28 Oct 2020)
- Do colliding neutron stars or supernovae produce heavy elements?:
The mystery of where heavy elements such as gold and silver come from has deepened with research groups clashing over whether binary neutron-star mergers can account for the abundance of those elements in the universe. On 17 August 2017 a burst of gravitational waves was detected by the LIGO and Virgo detectors. Astronomers quickly turned their telescopes towards the source of the waves and observed the afterglow of a kilonova – the collision of two neutron stars – in a galaxy 140 million light-years away. The light of the kilonova was powered by the radioactive decay of large amounts of heavy elements formed by rapid neutron capture (the “r-process”). In particular, a team led by Darach Watson at the Niels Bohr Institute at the University of Copenhagen identified the spectral line of strontium in the kilonova’s light.
- Enough collisions:
It is now certain that neutron-star collisions produce r-process elements such as strontium, europium, silver and gold. However, debate continues as to whether there are enough such collisions to produce the abundance of those elements that we observe in the universe.
In a paper submitted to Monthly Notices of the Royal Astronomical Society, astronomers led by Irina Dvorkin of the Institut d’Astrophysique de Paris studied how the interstellar medium becomes enriched in r-process elements and concluded that binary neutron-star mergers are the main source of these elements in environments that have generally low levels of heavy elements. These regions include the Milky Way’s halo, dwarf galaxies and the early universe. Although their models did not focus on environments richer in heavy elements, such as the Milky Way’s disc, they propose that binary neutron-star mergers could also be the dominant source there too.
One of their reasons for this conclusion is the observed variations in the ratios of r-process element abundances compared to iron-group elements, which are formed in supernovae. If r-process elements were also formed in supernovae, we would expect them to have a constant ratios relative to the iron-group elements. Instead, there is a large scatter observed in the ratios of the two element groups, implying different origins.
- Timescale too long:
However, this conclusion is disputed by a paper in The Astrophysical Journal by Chiaki Kobayashi of the University of Hertfordshire and team. Kobayashi and colleagues created models describing the origin of every element in the universe as a function of time and environment. They came to the conclusion that the timescale of binary neutron stars forming from supernovae and then spiralling into a collision is too long to explain the observed abundance of r-process elements.
“The important difference between our paper and the Dvorkin et al. paper is the time delay of neutron-star merger events,” Kobayashi tells Physics World. Following their formation, binary neutron stars could take billions of years to get close enough to collide. However, some binary neutron stars may be able to merge much faster; for example, PSR J0737-3039 – the only double pulsar discovered so far – is a relatively young binary neutron-star system that will merge within a timescale of 85 million years.
Dvorkin adds, “In my opinion, the fraction of fast mergers needs to be further studied, as it’s clearly central to the question of the early production of heavy elements.”
If a significant number of binary neutron stars can undergo fast mergers, it will speed up the rate at which they enrich the interstellar medium – the gas and dust between stars – with r-process elements.
Watson, who made the key strontium discovery in the kilonova afterglow, acknowledges that observations are currently contradictory. “The amount of material produced in small dwarf galaxies [with low heavy-element abundances] is, I believe, largely inconsistent with neutron star production,” he says. “However, I also believe that it may be possible for neutron stars to merge on fast timescales.”
- Sub-miocron diamonds:
Watson points out another line of evidence not considered in the Dvorkin or Kobayashi papers: nanodiamonds. These are tiny, sub-micron diamonds that can form in a variety of environments in space, from star-forming regions to asteroid collisions, but some nanodiamonds also contain r-process elements. Since neutron-star collisions do not produce nanodiamonds, the only other possible source of r-process enriched nanodiamonds is supernovae, says Watson.
Although the scatter in the ratios of r-process elements to iron-group elements observed by Dvorkin’s team seems to rule out ordinary supernovae, Kobayashi proposes a new type of exploding star called a magneto-rotational supernova. However, there is no direct evidence for the existence of such supernovae and the debate regarding the origin of r-process elements will continue to rumble on.
8. Physicsworld記事 (2020/10/28)
- 中性子星あるいは超新星の衝突は重金属を生成するか?:
金や銀のような重金属生成の謎は、連星の中性子星の合体が宇宙におけるこれらの金属量を説明できるという議論は観測結果と合わないという研究グループの指摘によって、議論が深化している。2017/8/17にLIGO (米国重力波観測レーザー干渉計)とVirgo (欧州重力波検出計)が重力波を検知した。天文学者は、直ぐ望遠鏡をその重力波発生の方向に向けて、140百万光年先のkilonova (中性子星の衝突による爆発現象)の残像を観測した。その残像であるkilonovaの光は、高速中性子捕獲による重元素合成(r-process)による多量の重元素の崩壊の際に生じる。特に、Copenhagen大学Niels Bohr研究所のDarach Watsonのチームがその観測を主導した。
- 十分な衝突があるか?:
今や、中性子星の衝突は、r-processによって、ストロンチウム、ユーロピウム、銀、金などの重金属を生成することが確かになっている。しかし、その量は我々が宇宙で観測する量を生成ほどの衝突があるのかについての議論が続いている。
Parisr天文物理研究所のIrina Dvorkinのチームは、Royal Astronomical Societyの月報に提出した論文では、恒星間の物質がr-processでどのように濃縮するかを研究し、連星の中性子星合体が、軽い方のレベルの重元素を含む環境生成の主な原因であると結論づけた。これらの地域には、我々の銀河のハロー、矮小銀河や初期宇宙が含まれる。このモデルではより重金属が濃縮する環境、例えば我々の銀河ディスクに焦点を当ててはいないが、連星の中性子星合体は、主要な原因となり得るとしている。
この結論の理由の一つは、超新星で生成される鉄グループ元素と比べてr-processで生成される元素の比率にばらつきがあることである。r-processによる元素が、超新星でも生成されるのであれば、我々は鉄グループ元素に比べて安定的な比率を得ることができるはずである。ところが、2つの元素グループの比率の値には大きなばらつきがある。
- 長過ぎるタイムスケール:
しかしこの結論に対しては、Hertofordshire大学のChiaki KobayashiによるThe Astrophysical Journalでの論文で議論になっている。Kobayashiとその同僚は、時間と環境の関数としての宇宙における各々の元素の成因を記述したモデルを作成した。彼等は、超新星から生成される連星の中性子星、その後、その連星が回転しながら合体に至るまでのタイムスケールは、r-processによる生成元素の観測される量を説明するには、長すぎるというのである。
"我々の論文とDvorkin他の論文との重要な相違点は、中性子星の合体という現象までの時間の遅れがある"とKobayashiはPhysics World誌で述べている。それら元素生成について、連星の中性子星が接近して衝突するまで数十億年かかる。しかし、一部の連星の中性子星はもっと早く合体し得る。今まで唯一発見された二重パルサーPSR J0727-3029の場合は、比較的若い連星の中性子星系で、今後85百万年のタイムスケールで合体するとされている。
さらにDvorkinが言うには、"私の意見では、早期の合体の割合をさらに研究する必要がある。それは重たい元素が早期に生成されるかという問題の核心でもあるからである。
"もし、連星系の中性子星の相当数が、早期に合体することができれば、恒星間のガスや塵物質のr-processによって濃縮する率のスピードアップになる。"
kilonovaの残像中に核となるストロンチウムを発見したWatsonは、現在の観測結果との矛盾は認識している。"(軽い方の重元素が多く含まれる)小さな矮小銀河で生成される元素量は、中性子星による生成と大きな不一致点がある"と私も思うとしている。"しかし、同時に中性子星の合体がもっと早いタイムスケールで起こる可能性もあり得る"と語った。
- サブミクロンのダイアモンド:
Watsonは、DvorkinやKobayashiの論文で触れていない別の証拠を指摘した。それは、サブミクロンという微小なダイアモンドが宇宙空間の様々な環境で生成され得るということで、恒星生成地域から小惑星が衝突する地域までの空間では、ナノ・ダイアモンドにはr-process生成元素も含まれるとしている。中性子星の合体では、ナノ・ダイアモンドは生成されず、ナノ・ダイアモンドがr-processで濃縮される可能性は、超新星しかないと、Watsonは言う。
Dvorkinチームが観測した鉄グループ元素に対するr-process生成元素との比率のばらつきは、通常の超新星を除外しているように見えるので、Kobayashiは、磁気回転型超新星と呼ばれる新しいタイプの恒星爆発を提案している。しかし、そのような超新星の直接的な証拠は見つかっておらず、r-process生成元素の起源に関する議論は今後も続くことになる。
>Top 9. Tracing gold's cosmic origin story, Proceedings of National Academy of Sciences of USA, Ken Croswell, 26 Jan 2021:
- Astronomers thought they had finally figured out where the gold, platinum, and other heavy elements in the universe came from. In light of recent results, they’re not so sure.
- Desperate phone calls made in the dead of night rarely convey good news, much less first word of a major scientific discovery. Alex Ji made such a call in 2015 from atop a mountain in Chile, where he was using one of the world’s largest telescopes. “This was actually the first time that I had taken data on a telescope ever,” says Ji, then a graduate student at the Massachusetts Institute of Technology in Cambridge. “I was absolutely convinced that I had done something wrong.”
- So, just after midnight, Ji called his advisor Anna Frebel to describe what he had just seen and could barely believe: A faint star in a dim nearby galaxy named Reticulum II seemed to have extraordinary amounts of europium, an element that’s even rarer in the cosmos than gold. Frebel told Ji to observe another star in the same small galaxy. That star also turned out to be rich in europium, as did a third. In the end, seven of the nine stars Ji observed had extreme levels of the rare element. It would take some time for the astronomers to interpret their surprising result, but when they did, it bore on one of the most pressing questions in astronomy: How does the universe make its heaviest elements?
- It’s a question astronomers have wrestled with for more than half a century. Elements such as gold, silver, and platinum don’t normally form in the nuclear reactions that power stars. So most researchers had long thought that these elements were created when massive stars explode at the ends of their lives as fiery supernovae.
- Ji’s 2015 discovery helped overturn that conventional wisdom. Two years later, a dramatic observation seemed to confirm the new thinking. Astronomers saw heavy elements actually forming when two dense neutron stars spiraled into each other, which implied that merging neutron star binaries and not supernovae were the main source of the heaviest elements.
- Now, however, doubts have arisen, suggesting that the original solution—supernova explosions—may be an important part of the answer after all.
- Rare Finds:
- These heavy elements barely exist. If you add up every atom in the universe from gallium (atomic number 31, which is the number of protons in the nucleus) to uranium (atomic number 92), you’d have only 1/2,300th of the total number of iron atoms (atomic number 26). Europium (atomic number 63) and gold (atomic number 79) belong in this same category.
- The heaviest elements are rare because stars hardly make them. To survive, every star must generate energy so as not to collapse under its own weight. This energy comes from nuclear fusion reactions initiated by intense heat and pressure. The reactions start as hydrogen fuses to form helium, which later gets transformed into heavier elements, such as carbon and oxygen. But these reactions produce energy only up to iron. Iron is a nuclear dead end because it is the most stable element: Fusing it to create heavier ones actually requires energy, which means stars don’t normally make them.
- Elements slightly heavier than iron, such as copper and zinc, can be forged in the turmoil of a supernova explosion. But what about even heavier elements? In the 1950s, astronomers and physicists identified two processes as the source of these elements (1, 2). One, called the s-process (“s” for slow), involves nuclear reactions near the end of a star’s life that release neutrons. Unlike protons, neutrons can sail into the positively charged nucleus of an atom without getting repelled. As a result, the iron nuclei the star inherited at birth occasionally capture these neutrons; because the neutron flux is low, the newly formed atomic nuclei have plenty of time to decay if they are radioactive. During this process, neutrons in the newly formed nuclei can turn into protons, thereby creating elements with greater atomic numbers. Astronomers have long observed s-process elements such as technetium on the surfaces of the aging stars that are making them.
- But the s-process didn’t explain everything. In particular, most gold, silver, and platinum, as well as all thorium and uranium, are synthesized when a rapid flux of neutrons bombards iron nuclei. New neutrons accumulate in nuclei before those captured earlier can decay, leading to even heavier elements. This is the r-process, as in “rapid.” It occurs in nuclear bombs, which is why for decades astronomers thought that supernova explosions drove the r-process and accounted for the universe’s gold and platinum. Whereas an aging star can spend millions of years churning out s-process elements, a supernova rams neutrons into iron nuclei and forges r-process material in mere seconds.
- The rapidity of the process, however, means that it’s much more difficult to study the r-process than the s-process. Until recently, no one had ever seen the r-process actually operate in space, and no one has ever seen a supernova create r-process elements.
- To study the r-process, astronomers have long focused on europium. Unlike gold, whose spectral lines lie in the ultraviolet region of the electromagnetic spectrum and are blocked by Earth’s atmosphere, europium’s spectral lines appear in the visible part of the spectrum. The high europium abundance that Ji found in the galaxy Reticulum II yields a key clue to the r-process (3): “Whatever produced this r-process element is very rare,” Ji says. In particular, it must be much rarer than an ordinary supernova.
- This conclusion follows from the nature of Reticulum II. It is an ultra-faint dwarf galaxy, with just a few tens of thousands of stars, and it is so dim that astronomers spotted it only a few years ago—even though it’s in our backyard, just 100,000 light-years from Earth. The nine stars Ji observed told the history of the numerous supernovae that had occurred in the galaxy. Two stars are extremely iron-poor, because they formed earliest, after only a few stars had exploded to supply the iron. These two iron-poor stars also lack europium. Then additional supernova explosions occurred, raising the level of iron in the galaxy and in stars that formed later. Somewhere along the way, a rare r-process event showered the galaxy with europium. The seven europium-rich stars Ji observed formed from this material, which also had higher levels of iron. Thus, whatever drove the r-process was a lot rarer than a typical supernova—so rare it had happened only once in Reticulum II and not at all in the previously studied ultra-faint dwarf galaxies.
- The rare r-process event might have been an exotic supernova. But Ji and his colleagues favored a different idea, one that another graduate student had explored four decades earlier.
- Star Turns:
- In 1973, James Lattimer was pursuing his doctorate in astronomy at the University of Texas, Austin. Although supernovae were then thought to be the source of r-process material, Lattimer’s advisor, David Schramm, suspected that these explosions might not actually produce the heaviest elements. So Schramm suggested that Lattimer look at something else: a neutron star, the tiny but super-dense core of a dead massive star. “Since I was a student at the time, I was more than happy to get paid to work on something that was exciting and kind of outlandish,” Lattimer says. “He suggested we try to find ways we could get neutron stars to blow up.” After all, if you want lots of neutrons to drive the r-process, a neutron star seems like a good bet.
- Another graduate student—Jocelyn Bell, in England—had discovered the first neutron star a few years earlier. Lattimer and Schramm calculated what would happen if a neutron star spiraled into a black hole. The black hole’s tidal forces would tear the neutron star apart, ramming neutrons into nearby iron nuclei and producing a profusion of gold, platinum, and other r-process elements. The amount made, their study showed, would be about 5 percent of a solar mass, plus or minus 5 percent of a solar mass. “Looking back, I laugh, because that kind of covers all the bases, including zero,” Lattimer says. Their article, published in 1974, argued that mergers between neutron stars and black holes could make all of the gold, platinum, and other r-process elements in the universe, eliminating the need for their creation in supernovae (4).
- It was also in 1974 that astronomers discovered the first neutron star binary: two neutron stars orbiting each other. Even before this discovery, Lattimer and Schramm recognized that the merger of two neutron stars could also make r-process material. That calculation was more complicated, however, and Lattimer never pursued it. Schramm later enlisted another student, Eugene Symbalisty, who confirmed the concept in the early 1980s (5).
- But the idea had little initial impact. Lattimer recalls one of his superiors saying that he “should have worked on a real problem rather than something so speculative.” After all, no one had ever seen two dead stars coalesce and create r-process material.
- Flash of Inspiration:
- All that changed on August 17, 2017. Astronomers detected signals from the merger of two neutron stars in a galaxy 130 million light-years away. The resulting gravitational radiation reached Earth (6), along with a burst of gamma rays (7) and—crucially—a flash of visible light (8): “The holy trinity all at once,” says Stan Woosley at the University of California, Santa Cruz.
- Woosley, a theorist, had long struggled to get supernova models to make r-process material, so he had already concluded that neutron star mergers were probably the solution. By observing the visible light of the 2017 merger, which came from the radioactive decay of the many r-process elements produced during the event, astronomers deduced the amount of material made: roughly 5 percent of a solar mass, in line with the predictions from Schramm and his students. It was the first time, and still the only time, that anyone had seen the r-process in action beyond Earth. Unfortunately, Schramm never lived to see the historic discovery; he died piloting a small plane in Colorado in 1997.
- The 2017 neutron star merger that confirmed Schramm’s idea convinced many astronomers that these events, once considered exotic and far-fetched, made nearly all of the gold, platinum, and other r-process elements in the universe. Ji, now at the Carnegie Observatories in Pasadena, CA, says he too thought that neutron star mergers were the answer, and he still leans that way, but he and others are also considering alternate theories. “I think most astronomers outside the field said, ‘Oh, great. This looks like the thing is all solved. Now it’s neutron star mergers,’” says John Cowan at the University of Oklahoma in Norman. “But I think the people working in the field realize there’s still some complications to solve” (9).
- For one thing, ever since the 1980s astronomers have seen europium in some of the Milky Way’s oldest stars, which formed soon after the birth of our galaxy. If neutron star mergers really made this europium, how did two neutron stars in a binary system spiral into each other so fast? The coalescence, which is thought to take roughly 100 million years or more, occurs because the system radiates gravitational waves, causing the neutron stars to slowly draw closer together.
- Astronomers are therefore taking another look at supernovae, because massive stars can explode just a few million years after their birth. If some of these supernovae managed to forge gold, europium, and other r-process elements, then stellar explosions could explain the presence of this material in the galaxy’s senior citizens.
- In fact, supernova explosions are a necessary part of the solution, says Chiaki Kobayashi at the University of Hertfordshire in England. Neutron star mergers “are not enough” to explain all the r-process material in the universe, Kobayashi says. She and her colleagues recently set out to understand the origin of every element from carbon to uranium. They calculated how stars with different lifetimes endow the galaxy with different elements, then compared the observed compositions of stars from young to old. For example, short-lived high-mass stars make lots of oxygen, so this element appeared early in the Milky Way’s history, whereas iron took longer to form, because most of that element comes from the explosions of long-lived stars. By comparing their models of the galaxy’s chemical evolution with actual observations of europium and other r-process elements in stars of different ages, Kobayashi’s team concluded that neutron star mergers made only a fraction of the universe’s r-process material; some rare type of supernova made the rest (10).
- Ironically, Kobayashi can’t explain the best-known r-process element: gold. “This is a really big mystery,” she says. Her calculations indicate that neither neutron star mergers nor supernova explosions make nearly enough gold.
- It’s possible that researchers have greatly overestimated the amount of gold in the universe. But the two different ways they determine the cosmic gold abundance—by observing the Sun and measuring meteorites—agree with each other. So Kobayashi says that the estimates of the nuclear reaction rates that make gold may be incorrect.
- Star Witness:
- Despite current uncertainties, some things now seem clear about the r-process. First, it’s rare. Whether neutron star mergers or some exotic supernovae mint gold, silver, and other r-process elements, these events happen infrequently, even in a galaxy as large as ours. The Milky Way has about two supernovae a century, but r-process events occur roughly a thousand times less often.
- Second, neutron star mergers made some of the gold and platinum on Earth and throughout the universe.
- Third, most supernova explosions make none. If, as Kobayashi says, supernovae also create r-process elements, it’s only a rare breed—less than 1% of all supernovae—that do so.
- Future discoveries could change the thinking again, especially the observation of a second neutron star merger that spawns r-process material. “It’s never good to base a whole paradigm on one observation,” Woosley notes. Seeing a second such merger would help pin down the frequency of these rare events and also how much r-process material each one produces.
- In addition, astronomers may someday find a pair of neutron stars so close together that they will merge in only a few million years. If that neutron star binary is young—for example, if it resides in a star-forming region—then it will mean that such binaries can form quickly and merge quickly, a sign that neutron star mergers might have occurred soon after the Milky Way’s birth. That would explain the presence of europium in the galaxy’s oldest stars and eliminate the need for supernovae to create the element.
- On the other hand, astronomers may succeed in seeing r-process material emerge from a supernova. Such a discovery would demonstrate that these stellar explosions did forge some of the most precious metals on Earth. But those observations will be difficult to pull off; the faint glow of supernova-born r-process elements would be hard to discern amidst the brighter light sparked by the creation of iron.
- The quest for a comprehensive understanding of the r-process sites in space will continue for some time. “I literally thought that three years ago we were basically done with this question,” says Ji, who now realizes things are more complicated than they had seemed in 2017. “We’re not done with this question, but I hope we figure it out in the next five years.” ❑
9. 宇宙での金の起源の物語, Ken Croswell, PNAS, 2021/1/26:
- 天文学者は、宇宙における金、白金など重金属の成因がいずれ解明されると考えている。まだ現状の結果では、わからないこともあるが。
- 真夜中の電話は悪いニュースが多く、良いニュースは少ないものだ。特に主要な科学上の発見の最初の時も特にそうだった。Alex Jiから、2015年に、チリにある世界最大級の望遠鏡を使用している山頂から電話がかかってきた。"今まで望遠鏡から得られたデータでは初めてかも知れない"と、 当時はMITの大学院学生だったJiは言った。"何か悪いことをしてしまったのかも知れないと思った"と。
- 真夜中を過ぎた後で、Jiは、アドバイザーのAnna Frebelを呼んで、彼が観測したことを記述してもらった。それを見ると全く信じられないことだった。つまり、Reticulum IIと呼ばれる近くの小さな銀河にある暗い星に異常な程のユーロピウム元素 (Eu-63)が存在しているように見えた。その元素は宇宙では金よりもずっと稀な金属である。その恒星はユーロピウムが3番目に豊富にあることがわかった。さらに、Jiが観測した9つの恒星の内、7つの恒星にも稀な金属元素が極端に多いことも観測できた。この驚くべき結果を天文学者が解釈するにはもう少し時間がかかり、その結果、天文学者の間で、今最も注目される話題を含んでいることになった。それはこの宇宙で重金属元素がどのようにしてできたかという問題である。
- それは天文学者がここ半世紀の間、取り組んできた問題だった。金・銀・白金などの元素は、恒星を輝かせている核反応では通常は生成されない。多くの研究者たちは、これらの元素は、激しい超新星として星の寿命が尽きる時の大爆発で作られるものを考えてきた。
- Jiの2015年の発見は、これまでの知識をひっくりかえすようなものだった。その2年後、また劇的な観察によって新たな知見を確信することになった。天文学者の考えでは、重金属が生成される主な原因は、超新星爆発ではなくて、中性子星の連星が相互に回転して合体衝突する時にできるではないかというのである。
- この見解によって、従来の超新星爆発が原因という理論が、主要な答えの部分ではないのではという疑問が生じてきた。
- 稀な発見:
- これらの重金属元素の存在量は極端に少ない。宇宙におけるガリウム(Ga-31)からウラニウム(U-92)全ての元素を合計しても、鉄元素(Fe-26)の1/2300にしかならない。ユーロピウム(Eu-63)と金(Au-79)は(周期律表の)同じ族に属する。
- 重金属元素の存在が稀なのは、星ではそれらを生成できないからである。恒星は、それ自身の重量でつぶれないようにエネルギーを生み出している。そのエネルギーは巨大な熱と圧力を生む核融合反応からきている。その最初の反応は水素が融合してヘリウムを作り、その後炭素や酸素のような、より重たい元素に変換していく。しかし、この反応は鉄までである。それ以上の重たい元素を生成するには、もっとエネルギーが必要となるが、恒星では通常はそこまで行かない。
- 鉄よりすこし重たい銅(Cu-29)や亜鉛(Zn-30)は、超新星爆発の時に作られる。それより重たい元素の場合はどうなるのか。1950年代に、天文学者や物理学者はこれらの元素生成の2つのプロセスを特定した。その一つは、Sプロセス(S=Slow) と言われ、恒星の寿命の終わりに中性子が放出される頃に作られる。中性子は、陽子とは異なり、+電荷を帯びた原子核の中に反発を受けないで侵入できる。その結果、恒星が誕生当初から元々持っていた鉄の原子核がこれらの中性子を吸収する。中性子の流入量は低いので、新たに作られた原子核は、放射性崩壊を起こす時間的余裕がある。このプロセスを通じて、原子核に侵入した中性子は、陽子に転換していき、それによって原子番号の大きな元素が生成される。天文学者はこのSプロセスによって、テクネチウム(Tc-43)が、年老いた恒星の周辺にできることを観察してきた。
- しかし、Sプロセスでは全てを説明しきれない。特に、金(Au-79)・銀(Ag-47)・白金(Pt-78)やトリウム(Th-90), ウラニウム(U-92)は、鉄の原子核に中性子が高速流入しない限り合成されない。原子核に獲得されて集積した新たな中性子は、放射性崩壊によってより重たい元素へ転換することができる。これがRプロセス(R=Rapid)と言われ、核爆弾の中で起こる反応である。このRプロセスを引き起こす超新星爆発は宇宙における金や白金の存在を説明できると、天文学者は何十年も前から考えてきた。年老いた恒星は何百万年もかけてSプロセスで元素を生成するのに対し、超新星は、鉄の原子核に落ち込んだ中性子を秒単位で新たな元素を生成する。
- このRプロセスは、プロセス反応があまりにも高速なので、Sプロセスより、研究するのが非常に難しい。今まで誰も、宇宙空間で実際にRプロセスを観測したことはなかったし、誰も超新星でRプロセスによる元素生成を観察した人はいなかった。
- Rプロセスを研究するために、天文学者は長い間、ユーロピウム(Eu-63)に着目してきた。金の場合のスペクトル線は電磁スペクトルの紫外線領域にあり、地球大気に阻まれていたが、ユーロピウムのスペクトル線は、可視光の領域にあり観測できる。Jiが、Reticulum II銀河で発見した豊富なユーロピウムは、このRプロセスを解明する鍵となる。"このRプロセスが生成した元素は非常に稀なはずで、特に通常の超新星でよりも稀な現象だ"とJiは語る。
- この結論はReticulum II銀河の特性からきている。それは極めて暗い矮小銀河で、恒星の数は数万個しかなく、あまりにも暗いので、地球から10万光年と近くにありながら、天文学者は、ほんの数年前までは注目してこなかった。Jiが観察した9個の恒星は、その銀河で起こった数多くの超新星の歴史を示しているという。2個の惑星は鉄量が極めて少なく、初期に生成されたことを示しており、数個の恒星爆発が鉄を供給したに過ぎなかった。これらの2個の鉄の少ない恒星はユーロピウムの量もまた少ない。それに引き続く超新星爆発で銀河内やそれ以降作られた恒星内の鉄量が増えた。これらの過程で、稀な現象であるRプロセスが銀河内のユーロピウム量を増大させた。Jiが発見した7個のユーロピウム豊富な恒星は、このような物質から形成されたので、鉄量も高い。Rプロセスは典型的な超新星よりはるかに稀な現象で、Reticulum II銀河でもただ1回しか起こらないほど稀なので、極めて暗い矮小銀河の研究も今まで行われて来なかった。
- 稀なRプロセス現象は、特異な超新星かも知れない。だが、Jiや彼の同僚は、別の考えを抱いている。それは40年ほど前に別の大学院生が研究したことでもある。
- 恒星は巡る:
- 1973年に、James Lattimer は、Austinのテキサス大学天文学の博士論文を研究していた。当時は、超新星がRプロセス物資の原因であると考えられていたが、Lattmerの指導教官のDavid Schrammは、これらの超新星爆発は実際には重金属元素を生成していないかも知れないという疑問を持った。そこでSchrammは、Lattimerに他を探すように言った。それが、巨大な恒星の寿命が尽きた後できる超高圧の核からなる中性子星である。Latimerが言うには、"当時まだ学生だったので、風変わりな研究に夢中で取り組むことができ、かつ給料ももらえるとは全く幸せだった"と。”今度は中性子星が吹き飛ぶような方法を見つけて見ようということになった。”そうして、多くの中性子星がRプロセスを生じるとすれば、中性子星は格好の研究対象となると思った。
- また別の英国の大学院研究生のJocelyn Bellは、その数年までに最初の中性子星をは発見していた。LattimerとSchrammは、どうしたら中性子星はブラックホールになっていくのか計算した。ブラックホールの潮汐力は中性子星をも引き裂いてしまい、中性子を近くにある鉄の原子核に押し込まれた結果、金・白金などがRプロセスによって合成される。その生成される量は、彼らの研究では、太陽質量の5%程度(±5%)と計算した。"今考えると、5%±5%とはゼロの場合も含むということだった"とLattimerは苦笑して言った。彼らの論文は1974年で発表され、中性子星とブラックホールの合体が金・白金その他Rプロセスで生成されるというもので、超新星が生成される必要性を排除したものだった。
- また1974年には、天文学者は最初の中性子星の連星、2つの中性子星が互いの周りを回転しているのを発見した。この発見より前に、LattimerとSchrammは、2つの中性子星の合体はRプロセス物質が生成される可能性を認識していた。この計算はさらに複雑で、Lattimerはそれを追求しなかった。Schrammは、後に、1980年代初期からこの考えを確認していた別の学生のEugene Symbalistyを指名した。
- しかし、当初はこの考えはうまく行かなかった。Lattmerは、彼の先輩を呼んで、Symbalistyは、そのような冒険的なものでなく現実的な課題に取り組むべきだと諭した。結局、誰も、2つの中性子星の合体やそこで作られるRプロセス物質を発見した人はいなかった。
- インスピレーションのひらめき:
- 2017年8月17に全く違うことが起こった。天文学者は、130 百万光年離れた銀河の中で2つの中性子星の合体からの信号を検知した。その重力波が地球にも到達し、γ線爆発に続いて可視光の閃光もあった。"聖なる三位一体のような現象だ"とSanta Cruzにあるカルフォルニア大学のStan Woosleyは言った。
- Woosleyは、理論研究者で、長い間、超新星がRプロセス物質を生成するモデルと格闘してきており、すでに中性子星合体がおそらくその答えであろうと結論づけていた。2017年の合体で観察された可視光は、多くのRプロセス原子がその現象で生成され放射性崩壊をする過程で発生したものだったので、天文学者は生成された元素量を推定できた。その量は太陽質量の約5%であり、丁度Schrammとその学生が予測した量と合っていた。それは初めての現象で、地球外でのRプロセス現象が観測されたのは、今まで唯一である。不幸なことに、Schrammは、この歴史的な発見時に生存していなかった。彼は1997年にコロラド州での小型飛行機操縦していて亡くなった。
- Schrammの考えを実証した2017年の中性子星合体は、多くの天文学者に、この現象は異常で大げさなものと思われていた現象だったが、それが宇宙での金・白金その他のRプロセス元素を生成したと再度考えるようになった。Ji は、現在はCA州PasadenaにあるCarnegie天文台にいるが、かれもまた中性子星合体が答えであろうとの考えに傾いているが、他の人は別の理論を検討している。"他の分野の天文学者は、この中性子星合体の現象で全て解決できると思われているが、まさにこの分野で取り組んでいる人々は、解決するにはまだ複雑なことが残っている"、とNormanにあるOklahoma大学のJohn Cowanは言う。
- 1980年代以降、天の川銀河の古い恒星の中にユーロピウムが観測され、それが我々の銀河誕生の直後に形成されたことがわかった。もし中性子星合体が本当にこのユーロピウムを生成したのなら、連星の2つの中性子星は、どのように互いの中にそれほど急速に落ち込んでいくのだろうか。その合体には、およそ1億年かそれ以上かかると考えられている。なぜなら連星系は重力波を放射し、中性子星が徐々に接近しているとされているからである。
- それゆえ天文学者は、再度超新星を見直すことになった。それは誕生から数百万年で爆発するからである。もしこれらの超新星がうまく金・ユーロピウムその他のRプロセス元素を生成するとすれば、超新星爆発によって、銀河の中の古い恒星内にあるこの物質の存在量を説明できるからである。
- 実際に、この解決には超新星爆発が必要な部分であると、英国Hertforshire大学のChiaki Kobayashiは言う。中性子星の合体だけではRプロセス原子量を全て説明するには"十分ではない"とKobayashiは言う。彼女は彼女の同僚と一緒に最近、炭素からウラニウムに至る全ての元素の起源について理解したことを発表した。彼らは、寿命の異なる恒星が銀河に様々な元素を与える方法を計算した。そして若い恒星から年老いた恒星で観察された元素恒星と比較した。例えば、短命の大質量の恒星には酸素量が多い。これは酸素は天の川銀河の歴史の初期に生成され、一方、鉄はもっと長く生成にかかるので、長寿命の恒星の爆発で生成されるとした。この銀河の化学的進化のモデルと、それぞれ異なる年齢の恒星内で観察されるユーロピウムその他のRプロセス生成元素と比較することで、Kobayashiのチームは、中性子星の合体は宇宙におけるRプロセス生成物質の一部分に過ぎないと結論づけた。一部の稀な超新星のタイプについては後述する。
- 皮肉なことに、もっとも良く知られたRプロセス元素である金についてはうまく説明できない。"それは本当に大きな謎である"と彼女は言う。彼女の計算では、中性子星合体で超新星爆発でも、いずれでも十分な量の金は生成されないのである。
- 研究者が、宇宙に存在する金の量を過剰に計算した可能性はある。しかし、宇宙における金の存在量は、太陽および隕石から別々の方法で計算されており、それらは一致している。Kobayashiが言うには、金の生成した原子核反応の率が正しくないのかも知れない、と。
- 恒星の証言:
- 現状まだ不確定な要素はあるが、Rプロセスについてかなり明らかになってきた。第一に、それは稀な現象であること。中性子星合体であろうと、あるいは異常な超新星が金・銀・その他Rプロセス生成元素を作ったとしても、我々の天の川銀河のような大きな銀河でも、これらは頻繁に起こる現象ではない。天の川銀河では1世紀の内に超新星は2個現れ、Rプロセスは1000年に1回あるいはそれ以下と見られている。
- 第二に、中性子星合体は、地球や宇宙での金・白金の一部を生成した。
- 第三に、多くの超新星爆発はこれらを何も生成しない。Kobayashiが言うには、超新星がRプロセス元素を生成するとすれば、それはかなり稀なケースで、全ての超新星の1%未満だろうとしている。
- 将来の発見はこの考えを再度変更するかも知れない。特に、二番目の中性子星合体とそれに伴うRプロセス元素の生成を観測することによってである。Woosleyは"一つの観測で全ての考え方を決めてかかるのは良くない"と言う。それで2番目の合体現象を観察することで、これら稀な現象の頻度とRプロセスでそれぞれ生成される量を特定するのに役立つ。
- さらに、天文学者はいつか、中性子星連星が非常に近接して、数百万年で合体するのを観測できるかもしれない。もしその中性子星連星が若くて、しかもそれが恒星形成領域にあれば、その連星は非常に早く合体することとなり、それは、天の川銀河誕生のすぐ後で起こった可能性を示している。これならば、銀河内の古い恒星内のユーロピウムの存在量を説明でき、またその元素を生成する超新星の必要性も除去できることになる。
- 一方で、天文学者はRプロセス元素が超新星によって生成される可能性も追求している。これが発見されれば、恒星爆発が、地球上の貴金属の一部を生成したことになる。しかしこれらの現象の観察は非常に難しい。超新星が生成するRプロセス元素のかすかな光の輝きを、鉄を生成する時の明るい閃光の中で観察することは非常に難しい。
- このように、宇宙におけるRプロセス現象の場を包括的に理解しようとすることはしばらく継続する。"私は文字通り3年前にこの疑問を考えてきた"と、Jiは言い、今は、2017年当時よりももっと複雑になってきているとの認識を示し"我々はまだこの疑問が解けていない。今後5年以内に解決できることを期待している"と語った。 ❑
>Top 10. Birth of Elements: Leverhulme Trust 20 May 2021
- Birth of elements:
Chiaki Kobayashi and her team perform cutting-edge galaxy simulations, following hydrodynamics, star formation and nucleosynthesis, thus predicting the periodic table of the Universe.
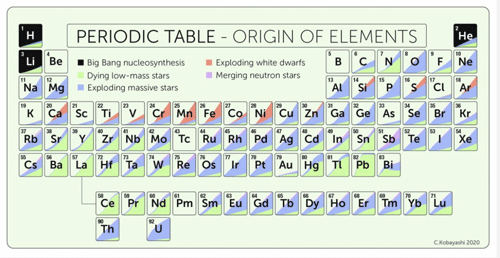
- Explaining the origin of the elements is one of the scientific triumphs linking nuclear physics with astrophysics. At the beginning of the Universe – the Big Bang – only light elements such as hydrogen and helium were produced. Heavier elements such as carbon, oxygen, iron and gold were instead created inside stars. As Carl Sagan said, “human beings are made of star dust”. But what about mobile phones? Very heavy elements beyond iron, such as gold and palladium found in our phones, need more exotic astronomical objects to be synthesised – perhaps a merger of two neutron stars and/or highly magnetised spinning massive stars. I have been calculating the production of elements following the evolution of the Universe and my theoretical prediction is shown in the periodic table of chemical elements above.
- At the beginning of the Universe there was no structure but only tiny density fluctuations. The Universe is made of 69% dark energy, 26% dark matter and just 5% normal matter, atoms, from which stars and galaxies formed. Over the next 13.8 billion years galaxies grow following the clustering of dark matter halos, stars are born and die in galaxies producing heavy elements there. Therefore, stars are fossils that retain the history of their host galaxies. At the end of their lives some explode as supernovae producing heavy elements that are distributed into the surrounding interstellar gas. New stars created from this gas contain elements produced by the previous generations of stars. By analysing the abundance patterns of the elements, it is therefore possible to determine how many and what kind of supernovae exploded in the past. I have been using this method – galactic archaeology – to study the origin of elements and the evolution of galaxies.
- Since various complicated astrophysical processes are involved, I have been using a super-computer to predict the chemical evolution of the Universe, by reproducing available observational data. In our Milky Way Galaxy, detailed elemental abundances of millions of stars are measured with recent observational surveys on several telescopes. It is not possible to resolve individual stars in external galaxies, but some chemical abundances can be measured for nearby to distant galaxies. In this project I will predict the origins and spatial distributions of elements in the Universe across cosmic time, beyond the reach of even the best telescopes that we currently have.
10. 元素の誕生: Leverhulme Trust誌 2021/5/20:
- 元素の誕生:
小林千晶と彼女のチームは、流体力学、星形成、核合成に続いて、最先端の銀河シミュレーションを行い、宇宙でのの周期表を予測している。
- 元素の起源を解明することは、原子核物理学と宇宙物理学を結びつける科学的勝利の一つである。宇宙の始まりであるビッグバンでは、水素やヘリウムなどの軽い元素しか作られなかった。炭素、酸素、鉄、金などの重い元素は、星の中で作られた。カール・セーガンが言うように、"人間は星の塵でできている"のだ。では、携帯電話はどうだろう。携帯電話に使われている金やパラジウムのように、鉄以外の非常に重い元素を合成するには、より風変わりな天体が必要である。例えば、2つの中性子星の合体や、高度に磁化された回転大質量星などが考えられる。私は、宇宙の進化に伴う元素の生成を計算して、その理論的予測を上記の元素の周期表に示す。
- 宇宙の始まりには構造はなく、小さな密度のゆらぎがあるだけだった。宇宙は、69%のダークエネルギー、26%の暗黒物質、そしてわずか5%の通常の物質である原子で構成されており、これらの物質から星や銀河が形成された。その後、(ビックバン以降) 138億年の間に、暗黒物質のハローが集まって銀河が成長し、その銀河の中で星が生まれて死んでいき、その度に重元素が生成されている。星はそれが属する銀河の歴史を保持している化石でもある。星の寿命が尽きると、一部の星は超新星爆発を起こし、重元素を生成して周囲の星間ガスに撒き散らす。この星間ガスから生まれた新しい星には、前の世代の星が生成した元素が含まれている。従って、元素の存在量のパターンを分析することで、過去にどのような種類の超新星がどれくらい爆発したかを知ることができる。私はこの方法 (銀河考古学) を用いて、元素の起源や銀河の進化について研究している。
- 複雑な天体物理学的プロセスが関与しているので、私はスーパーコンピュータを使って、入手可能な観測データを再現し、宇宙の化学進化を予測してきた。我々の天の河銀河では、いくつかの望遠鏡を使った最近の観測で、数百万個の星の詳細な元素組成が測定されている。外部の銀河では、個々の星を分解することはできないが、近くの銀河から遠くの銀河まで、いくつかの化学組成を測定することができる。このプロジェクトでは、私たちが現在持っている最高の望遠鏡でさえも到達できない宇宙の時間を超えて、宇宙における元素の起源とその空間分布を予測している。
>Top 11. Why the supergiant star Beterlgeuse went mysteriously dim last year, Nature, 16 June 2021:
- High-resolution images suggest the star spewed out so much dust that its brightness dropped by two-thirds in 2020.
- Last year’s dramatic dimming of the star Betelgeuse — familiar to many as the ‘right shoulder’ of the constellation Orion — was caused by a cloud of dust spewed out by the star itself. Astrophysicists reached this conclusion, published on 16 June in Nature1, using high-resolution imaging of Betelgeuse before and after the dimming, combined with computer simulations.
- Normally, Betelgeuse is one of the ten brightest stars in the night sky. For decades, researchers have known that it undergoes cycles of dimming roughly every 425 days, during which it temporarily loses about one-quarter of its peak brightness. But in February 2020, astronomers noticed that the star’s brightness had dropped by an unprecedented two-thirds — enough to be noticeable with the naked eye.
- The unexplained dimming fuelled speculation that the star could be about to explode. Betelgeuse is a red supergiant — a type of star that’s more massive and thousands of times shorter-lived than the Sun — and it is expected to end its life in a spectacular supernova explosion sometime in the next 100,000 years. This event would provide a spectacle the likes of which Earthlings have not seen in centuries: the last supernova in the Milky Way that could be observed from Earth was in 1604, and Betelgeuse is so close to our planet that its supernova will be bright enough to be visible during daytime for weeks. The star is around 168 parsecs (548 light years) away, according to the most current estimates.
- But many astrophysicists warned that the supernova speculation was wishful thinking. They pointed out that the dimming was likely to be caused by more mundane mechanisms, such as a blob of unusually cold matter appearing on the surface of the star in what’s known as a convective cell, or a cloud of dust crossing the line of sight to it.
- Now, astrophysicist Miguel Montargès at the Paris Observatory and his collaborators have found that the reason for the ‘great dimming’ was probably a combination of both of those factors.
- Mystery solved:
- The team took a series of high-resolution images of the star in January 2019, December 2019, January 2020 and March 2020, using the Very Large Telescope in Chile’s Atacama Desert. Betelgeuse is more than 16 times the mass of the Sun, and 764 times its width — so big that if it were located at the centre of the Solar System, it would engulf the orbits of all the planets up to Mars. This makes it one of the few stars that astronomers can resolve as a disk, rather than as a single dot of light, explains Montargès, who has been studying Betelgeuse on and off for a decade and has been interested in it since he was ten years old, when it was the first star that he was able to identify in the sky.
- The images clearly showed that the bottom left-hand part of the star — as seen from Earth's Northern Hemisphere — had dimmed dramatically, and that the position of the darker region did not change substantially over the imaging period. This indicated that the dim spot was caused by a cloud of dust that had been spewed by the star itself, and was moving roughly in the direction of the line of sight, rather than passing by. “If it had been one cloud transiting, it should have crossed the star,” Montargès says.
- The team’s explanation for the dimming is that an unusually cool convective cell led to a drastic drop in temperature in the star’s atmosphere. This enabled gas that the star had spewed out in the previous year to condense quickly into dust, blocking out light from the star. This scenario was the one that fit the data best, as the researchers confirmed by running more than 10,000 computer simulations. “The conclusion from the modelling is that both events happened at the same time,” says Montargès.
- “The fact that a simulation was run makes me more convinced” that the explanation is correct, says Meridith Joyce, an astrophysicist at the Space Telescope Science Institute in Baltimore, Maryland.
- “It would be wonderful if we could know in how many days or years Betelgeuse should explode as a supernova,” says astrophysicist Chiaki Kobayashi at the University of Hertfordshire in Hatfield, UK. However, researchers don’t yet have a sufficiently detailed understanding of Betelgeuse — or of red supergiants in general — to be able to make such a prediction.
11. 超巨星Beterlgeuseが、昨年、不思議と薄暗くなった理由、Nature、2021/6/16
- 高解像度の画像は、星が大量のほこりを吐き出し、2020年にはその明るさが2/3に低下したことを示唆している。
- 昨年、オリオン座の右肩として多くの人に親しまれている星ベテルギウスの劇的な減光は、星自体から噴出された塵の雲によって引き起こされた。 天体物理学者は、この結論に達し、6月16日にNature誌で公開された。これは減光前後のベテルギウスの高解像度画像とコンピューターシミュレーションを組み合わせて得られたものである。
- 通常、ベテルギウスは夜空で最も明るい10個の星の1つである。 何十年もの間、研究者はそれがおよそ425日ごとに調光のサイクルをすることわかっていた。それは一時的にそのピークの明るさの約1/4分を失う。しかし、2020年2月、天文学者は、星の明るさが前例のない2/3に低下したことに気づいた。これは肉眼でも十分に目立つほどである。
- 原因不明の調光は、星が爆発しそうだという憶測を煽った。 ベテルギウスは赤色超巨星であり、太陽よりも質量が大きく(16.5-19M⦿)、寿命が数千倍短いタイプの星であり、今後10万年以内に壮大な超新星爆発でその寿命を終えると予測されている。 このイベントは、地球人が何世紀にもわたって見たことのないような光景を提供する。地球から観測できた天の河の最後の超新星(SN1054) は1604年であり(6300ly)、ベテルギウスは私たちの惑星に非常に近いため、その超新星は十分に明るくなり、数週間、日中でも見えるようになる。 最新の推測では、この星は約168パーセク (548光年)離れている。
- しかし多くの天体物理学者は、超新星の推測は希望的観測であると警告した。 彼らは、調光が引き起こされるのは、よりありふれたメカニズムによって引き起こされる可能性が高い、即ち、対流セルとして星の表面に現れる異常に冷たい物質の塊や、視線を横切る塵の雲などを指摘した。
- 現在、パリ天文台の天体物理学者MiguelMontargèsと彼の共同研究者は、大きな調光の原因は、おそらくこれらの両方の要因の組み合わせであること指摘した。
- 謎の解明:
- チームは、チリのアタカマ砂漠にある超大型望遠鏡を使用して、2019/1、2019/12、2020/1、2020/3に星の一連の高解像度画像を撮影した。 ベテルギウスは太陽の16倍以上の質量で、その直径は764倍もある。これは非常に大きいので、太陽系の中心に位置すると、火星までの惑星軌道を飲み込むことになる。 Montargèsは説明によると、天文学者が1つの光の点としてではなく、円盤として分解できる数少ない星の1つとして、10年前から興味を持ってベテルギウスのオンとオフを10年間研究している。
- 画像は、地球の北半球から見た星の左下部分が劇的に暗くなったこと、そして暗い領域の位置が画像化の期間中に実質的に変化しなかったことを明確に示した。 これは、薄暗いスポットが、星自体が噴出した塵の雲によって引き起こされ、通り過ぎたのではなく、視線の方向に大まかに動いてたことを示している。"もしそれが1つの雲の通過だったとしたら、それは星を通り過ぎた
<通り過ぎた>通り過ぎた>
はず"とMontargèsはいう。
- 調光についてのチームの説明は、異常に冷たい対流セルが星の大気温度の劇的な低下をもたらした。 これにより星が前年に噴出したガスが急速に凝縮して塵になり、星からの光を遮断することになった。 研究者が10,000を超えるコンピューターシミュレーションを実行して確認したように、このシナリオはデータに最もよく適合するシナリオであった。"モデリングからの結論は、両方のイベントが同時に発生した"、とMontargèsはいう。
- Maryland州BaltimoreにあるSpace Telescope science Instituteの天体物理学者であるMeridith Joyceは、"シミュレーションが実行されたことによって、説明が正しいことを確信した" と述べている。
- "ベテルギウスが超新星として爆発する日数や年数を知ることができれば素晴らしいと思う"と、英国Hatfieldにあるhaertfordshire大学の天体物理学者小林千晶はいう。 しかし、研究者は、ベテルギウスあるいは一般的に赤色超巨星について、そのような予測を行う十分な詳細な理解をまだ持っていない。
>Top 12 Thank exploding stars for our galxy's gold; UK Research and Innovation, 7 July 2021:
- All the elements on Earth, from those that create humans to the air and even the jewellery we wear, all come from cosmological phenomena like exploding stars.
- Scientists have now discovered a new type of cosmic explosion that is likely to have created elements, such as oxygen, iron and gold, in our galaxy’s earliest stars. Meaning, the gold jewellery you own may be made of star dust as old as the earliest era of the Milky Way.
- Science worth its weight in gold:
- The study found evidence that one of the most powerful explosive events in our universe produced heavy elements in the earliest era of the Milky Way. The discovery was made using the SkyMapper Telescope in Australia. The event, called a magnetorotational hypernova, is a spinning, super-energetic supernova created by the collapse of a massive star.
- Dr Chiaki Kobayashi, co-author and Reader in Astrophysics at the University of Hertfordshire, said: "We would like to understand where we come from. The elements which compose our human bodies come from stars. But what about mobile phones, which contains precious metals such as copper, silver, and gold? Ancient stars we can observe only with big telescopes are amazing as they have recorded the history of the universe like fossils in archaeology. The discovery of this star is proof that these elements come from an explosion of a massive star, probably 25 times more massive than the sun, about 13 billion years ago."
- Jwellers should thank their lucky (dead) stars:
- The research conducted by Dr Kobayashi was supported by the Science and Technology Facilities Council (STFC), part of UK Research and Innovation. It was supported through a nuclear astrophysics consortium Bridge the Disciplines related to the Galactic Chemical Evolution.
- The STFC-supported study follows on from pioneering analysis international team of female astrophysics in 2020 (University of Hertfordshire). The all-female team led by Dr Kobayashi analysed the origins of all the elements in the periodic table. Their research concluded that spinning supernovae are most likely to be responsible for creating the abundance very heavy elements such as gold in the cosmos, rather than neutron star collisions as previously believed. This type of hypernovae also produced the light elements formed during massive star evolution and the iron-peak elements generated by explosive nuclear burning.
- Furthuer information:
- View the research paper: r-Process elements from magnetorotational hypernovae (Nature).
- The discovery of the precious star, known as SMSS J200322.54-114203.3, was made using the SkyMapper Telescope. This was led by co-authors David Yong and Gary Da Costa from Australian Research Council Centre of Excellence in All Sky Astrophysics in 3 Dimensions, at the Australian National University. The elements were measured by the 6.5-m Magellan telescope at the Las Campanas Observatory in Chile and European Southern Observatory’s Very Large Telescope.
12. 我々の銀河にある金のために爆発する星に感謝, UK Reseach and Innovation, 2021/7/7
- 地球上のすべての要素は、人間を作り出すものから空気や我々が身につける宝石まで、すべて、爆発する星のような宇宙現象から来ている。
- 科学者は今や我々の銀河の最も初期の星に酸素、鉄、金などの元素を作り出した新しいタイプの宇宙爆発を発見した。 即ち、あなたが所有する金の宝飾品は、天の河銀河の初期の時代と同じくらい古い星の塵でできているということになる。
- 金にはその重量に相当する科学的価値がある。
- その研究では、我々の宇宙で最も強力な爆発現象の1つが、天の河銀河の初期の時代に重元素を生成したという証拠を発見した。 この発見は、オーストラリアのSkyMapper望遠鏡を使用して行われた。 この現象は、磁気回転超新星と呼ばれる巨大な星の崩壊によって形成された回転する超エネルギー超新星である。
- Hertfordshire大学の共著者で天体物理学准教授である小林千晶博士は、次のように述べている。 "我々はどこから来たのかを知りたい。私たちの人体を構成する要素は星から来ている。銅・銀・金などの貴金属を含む携帯電話はどうだろうか?大きな望遠鏡でしか観測できない古代の星が、考古学の化石のように宇宙の歴史を記録していることは驚くべきことである。この星の発見したことは、これらの元素が、おそらく太陽の25倍の大きさの巨大な星の約130億年前の爆発に由来することの証拠である。"
- 宝石屋は彼らの幸運な(死んだ)星に感謝すべき:
- 小林博士による研究は、英国研究技術革新機構の一部である科学技術施設研究会議 (STFC)の支援を受けて行われた。 これは、銀河化学進化に関連する分野の橋渡しである核天体物理学コンソーシアムを通じて支援された。
- STFCが支援する研究は、2020年の女性天体物理学の先駆的な分析国際チーム (Hertfordshire大学)に続くものです。 小林博士が率いる全女性から成るチームは、周期表のすべての元素の起源を分析した。 彼らの研究は、回転する超新星が、それまで信じられていた中性子星の衝突ではなく、宇宙に金などの非常に重い元素を豊富に作り出す原因である可能性が最も高いと結論付けた。 このタイプの極超新星はまた、大規模な星の進化の間に形成された軽い元素と、爆発的な核燃焼によって生成された鉄をピークとする元素を生成した。
- さらなる情報:
- 研究論文参照:r-磁気回転極超新星からのプロセス元素 (Nature誌)。
- SMSS J200322.54-114203.3として知られる貴重な星の発見は、SkyMapper望遠鏡を使用して行われた。 これは、オーストラリア国立大学の全天天体物理学におけるオーストラリア研究評議会Center of Excellenceの共著者であるDavid YongとGary Da Costaが主導した。 また元素は、チリのLas Campanas天 文台にある6.5mのマゼラン望遠鏡とヨーロッパ南天天文台の超大型望遠鏡によって測定された。
>Top 13. The 2024 George Darwin Lectureship Award: (2014/1/14)
- Royal Astronomical Society, UK ():
- The 2024 George Darwin Lectureship is awarded to Professor Chiaki Kobayashi
- Professor Chiaki Kobayashi is an internationally recognised leader in the field of chemical evolution of galaxies, and a pioneer in the study of the origin of the elements – a subject which bridges nuclear physics and astrophysics.
Her best-known works on the topic include the “astronomer's version” of the Periodic Table which takes the well-known version of the Periodic Table and enriches it to provide for each element the cosmic abundance as a function of age of the Universe, listing each of the processes that led to their creation.
- Another recent highlight is the prediction of magnetorotational hypernovae being an additional site for the rapid neutron capture process (in addition to neutron star mergers), which has recently been confirmed by the discovery of a star with the expected chemical signature.
- This work has a direct impact on the predicted number of neutron star mergers that can be detected through their gravitational waves.
Professor Kobayashi is also a highly-experienced science communicator, with her work featuring in several news articles, and TV and radio programs across the globe.
For these reasons Professor Kobayashi is awarded the George Darwin Lecture.
2024年度 George Darwin Lectureship賞: (2024/1/14)
- 2024年ジョージ・ダーウィン講師賞を小林千晶教授に授与。
- 小林千晶教授は、銀河系の化学的進化の分野で国際的に認められたリーダーであり、元素の起源に関する研究での先駆者である。そのテーマは核物理学と天文物理学との架け橋となるテーマである。
彼女の一番著名な研究成果は、周期律表の"天文学者版"というテーマを含むものであり、それは有名な周期律表において各元素の生成プロセスをリストアップし、宇宙年齢の関数としての宇宙での存在量を示したことになる、
- さらに最近のハイライトとしては、磁気的回転を行う超新星の存在を予測し、そこでは急速な中性子捕獲プロセスが(中性子星の合体に加えて)行われているとした。そのことは最近、その化学的特徴をもつ恒星が発見されたことで確認された。
- これらの研究成果は、重力波の検知によって中性子星の数の予測をする上で直接的なインパクトがある。
なお、小林千晶教授は、科学を広く伝導する上で多くの経験を有しており、彼女の研究については、世界中で、幾つものニュース論文、テレビやラジオ番組で発表されている。
これらの理由により小林教授にジョージ・ダーウィン賞を授与するものである。
Comment
- It is interesting to know how the elements were formed by researching the mostly distant galaxies, furthermore which will eventually give us important hints how life or ourselves were born.
- I studied geology at the university, which had been additional field of science after physics, chemistry and biology. After a while, geological department has been merged with geophysical science, becoming one of major frontier of science covering environment of earth and solar system, and now having more crossover field with astronomy.
- Gold, or Au (atomic number 79) usually occurs in free elemental form (native gold), which is relatively rare element (abundance ration in the earth crust around 0.003 ppm. Usual gold mine produces 1-5 g/ton. Until now, human being has mined gold about 180K tons, mining about 3,000 tons annually, which will dig up in a couple of decades. Human deep attachment or obsession of gold has dug or squeezed thoroughly; thus making to look apparently more abundant gold than its real existence on the earth.
- 最も遠方の銀河を調査することで元素の生成を知ることができるのは興味深い。そしてさらにそのことが生命や人間がどうして生まれたのかの重要なヒントを与えてくれる。
- 私は大学で地質学を学んだが、それは物理・化学・生物に続く追加的な科学分野だった。しばらくして地学科は地球物理学科と合併となり、地球や太陽系の環境をカバーする主要なフロンティアの一つとなった。そして今や天文学の分野ともますます重複する分野が増えている。
- 金 (元素番号79)は、通常自然金の形で産出する。金は、地球の地殻には、約0.003 ppmしか存在しない。通常の金鉱山は金含有量で1-5g/トンである。今まで人類が採掘した金の量は約18万トンで、毎年3千トンを採掘しており、あと20-30年で枯渇すると言われる。人間の金に対する愛着や執念が、これまで徹底的に採掘してきた。その結果、金はその稀な存在比に関わらず、見かけ上豊富にあるように見せているのではないか。
Origins of the elements in the universe |
Cat: SCI |
|
Chiaki Kobayashi, et.al. |
20923u/1710r |
Original resume |
Remarks |
>Top 0. Preface:
|
0. 序文:
|
>Top 1. University of Hertfordshire (UK), UH, , 16 Sept 2020
|
1. University of Hertfordshire (UK):
|
>Top 2. Science Alert: Michelle Starr, 15 Sept. 2020
|
2. Science Alert:誌の記事:
|
>Top 3. The Daily Galaxy, Great Discoveries Channel, Posted on Sep. 15, 2020
|
3. The Daily Galaxy誌の記事: 偉大な発見経路:
|
>Top 4. Notimérica: Ciencia-Tecnología, Madrid, 15 Sep., 2020:
|
4. Notimérica: Science-Technology:
|
4. Notimérica: Ciencia-Tecnologia誌の記事:
|
>Top 5. VICE: Becky Ferreira, 18 Sept. 2020:
|
5. VICE: Becky Ferreira:
|
>Top 6. Cf: Type Ia Supernova: (SNIa) (by Wikipedia)
|
6. <参考>: Ia型超新星: (Wikipedia)
|
>Top 7. Cf: Distance measurement by Type Ia Supernova: (Hubblesite)
|
7. <参考>: Ia型超新星による距離測定: (Wikipedia)
|
>Top 8. Physicsworld (28 Oct 2020)
|
8. Physicsworld記事 (2020/10/28)
|
>Top 9. Tracing gold's cosmic origin story, Proceedings of National Academy of Sciences of USA, Ken Croswell, 26 Jan 2021:
|
9. 宇宙での金の起源の物語, Ken Croswell, PNAS, 2021/1/26:
|
>Top 10. Birth of Elements: Leverhulme Trust 20 May 2021
|
10. 元素の誕生: Leverhulme Trust誌 2021/5/20:
|
>Top 11. Why the supergiant star Beterlgeuse went mysteriously dim last year, Nature, 16 June 2021:
|
11. 超巨星Beterlgeuseが、昨年、不思議と薄暗くなった理由、Nature、2021/6/16
|
>Top 12 Thank exploding stars for our galxy's gold; UK Research and Innovation, 7 July 2021:
|
12. 我々の銀河にある金のために爆発する星に感謝, UK Reseach and Innovation, 2021/7/7
|
>Top 13. The 2024 George Darwin Lectureship Award: (2014/1/14)
|
2024年度 George Darwin Lectureship賞: (2024/1/14)
|
|
|
|
|
Comment |
|
|