>Top 3. Lithium-ion (LIB) Battery:
- The tremendous growth in high-power portable electronic devices (cellular phone, note PC, and video recorder) has increased the demand for lightweight.
- features: high density, no memory effect, low self-discharge, and safety risks when damaged.
- $Li^{+}$ move from the negative electrode to the positive electrode during discharge.
- $Li^{+}$ battery uses an intercalated Li compound as one electrode material, compared to the metallic Li used in a non-rechargeable Li battery.
- mostly based on $LiCoO_2$
- $LiFePO_4; LiMn_2O_4$, and $Li_2MnO_3 (LMO)$, and $LiNiMnCoO_2 (LMC)$ are lower energy density but longer lives and less likelihood of fire or explosion.
- $LiNiCoAlO_2$ (NCA) and $Li_4Ti_5O_{12}$ (LTO) for particular niche roles.
- >Top Until recently, the most common rechargeable battery was Ni-Cd (nicad) battery.
- The electrode reactions are the following:
- Cathode: $2NiO(OH)+2H_2O+2e^{-} \rightarrow 2Ni(OH)_2+2OH^{-}$
- Anode: $Cd+2OH^{-} \rightarrow Cd(OH)_2+2e^{-}$
- A single nicad voltaic cell has an emf of 1.30V; typically contain more cells in series to produce higher emfs.
- But Cd is a toxic heavy metal; rough 1.5B nicad batteries are produced annually.
- Development of $NiMH$ battery:
- Anode consists of a metal alloy, such as $ZrNi_2$, that has the ability to absorb $H_2$ atoms.
- During oxidization at the anode, $H_2$ atoms are released as $H_2O$
- >Top The newest rechargeable battery to receive large use in consumer electronic (and EV), Li-ion batteries achieve a greater energy density.
- Li-ion battery achieves a greater energy density than Ni-based battery.
- Li-ion can be inserted into layers of graphite.
- During discharge Li-ions migrate between two different layered materials that serve as the anode and cathode of the cell.
- History of development of LIB:
- 1973: Adam Heller proposed lithium thionyl chloride battery.
- 1977: Samar Basu demonstrted electrochemical intercalation of $Li$ in graphite.
- 1979: Ned A. Godshall, and in 1980 John Goodenough and Koichi Mizushima both demonstrated a rechargeable lithium cell in 4V using $LiCoO_2$ as the positive electrode and $Li$ metal as the negative electrode.
- 1980: Richard Yazami demonstrated the reversible electrochemical intercalation of $Li$ in graphite.
- 1982: Godshall et al. were awarded US Patent on the use of $LiCoO_2$
- 1983: Michael Thackeray, John Goodenough et al. further developed manganese spinel as a positive electrode.
- 1985: Akira Yoshino assembled prototype cell using carbonaceous material into which $Li^{+}$ could be inserted as one electrode, and $LiCoO_2$ as the other. By using materials without metallic $Li$, safety was dramatically improved. $LiCoO_2$ enabled industrial-scale production.
- Commercial production:
- 1991: Sony and Asahi Kasei released first commercial LIB.
- 2011: LIB accounted 66% of all portable secondary battery sales in Japan.
- 2012: John Goodenough, Rachid Yazami and Akira Yoshino received 2013 IEEE medal for environmental and safety technologies of LIB.
- 2014: Then National Academy of Engineering recognized John Goodenough, Yoshio Nishi, Rachid Yamami and Akira Yoshino for their pioneering efforts in the field.
- 2016: Global LIB production capacity was 28GWh, with 16.4 GWh in China.
- >Top Tesla's Gigafactory ($5B):
- Panasonic reached agreement with Tesla to invest $1.6B in a factory, leading battery cell production of manufacturing.
- Production Group of LIB:
- Tesla/Panasonic Group
- Nissan/AESC (Nissan+NEC) Group
- GM/VW/LG Chem Group
- Mitsubishi/GS Yuasa/Toshiba Group
- Gigafactory-1: Reno, Nevada, US;
- Tesla held a grand opening on 2016/7/29, having only 3/21 blocs completion of the Gigafactory; which began mass production in 2017/1; expected to be completed by 2020.
- the factory is aligned on true north, is designed to be energy self-reliant (solar, wind, and geothermal), largest footprint in the world.
- Model-3 production ramped up to about 5,000
- Gigafactory-2: Buffalo, New York
- Gigafactory-3: Shanghai, China; began construction
- Gigafactory-4?: in Europe, plans underway
- EV Battery production <Fig.>:
- CATL (Comtemporary Amperex Technology, 宁德时代新能源科技): having plan to produce 88GWh by around 2020.
- BYD Company Ltd. (比亚迪股份): will produce 60GWh in 青海省 by 2020; particulary higher energy density battery of NMC811 (Ni8-Mn1-Co1 cathode) than MNC622 (Ni6-Mn2-Co2)
- it is importnat to decrease use of rare metal Co from 2 to 1.
- Co is said as 'the Wall of EV Proliferation', which is mostly mined unstable DRC (Democratic Republic of Congo)
- Tesla aims to reduce Co in producing NCA (Ni-Co-Al) battery using only 3% of Co.
|
3. リチウムイオン電池:
- energy density: エネルギー密度 Wh/kg, or Wh/m3
- ramp up: increase the level
- electrolyte: 電解質
- thionyl: チオニル基
- NEV: 新能源车
|
- Li-ion Battery (LIB) structure:
- Positive electrode: $LiCoO_2; LiMn_2O_4; LiFePO_4$
- Negative electrode: $C; Si; SnO_2$
- Electrolyte: $LiPF_6; LiClO_4$
- AA Size (L) and 18650 for Tesla Model-S; Tesla says that now new 21700 type battery with 70mm-L, 21mm-D & 24.245mm3, which is bigger (46%), improved energy efficiency about 15%, energy density 877.5Wh/L and cell capacity 21.275Wh than 18650 type battery.
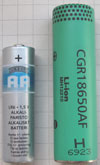
- Tesla's Gigafactory-1:
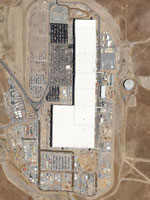
- EV Battery Production Share:
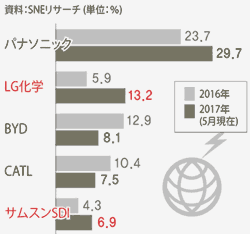
|
>Top <Next Generation Cathode - NMC 811>
- Various Li-ion Batteries:
-
$LiCoO_2$ |
LCO |
MobilePhone, Laptop PC, Camera |
$LiMn_2O_4$ |
LMO |
Elec tool, Medical, Hobby |
$LiFePO_4$ |
LFP |
Elec tool, Medical, Hobby |
$LiNiMnCoO_2$ |
NMC |
Elec tool, Medical, Hobby |
$LiNiCoAlO_2$ |
NCA |
EV, Gridstorage |
$Li_4Ti_5O_{12}$ |
LTO |
EV, Gridstorage |
- NMC (Ni-Mn-Co) Cathode:
- This is the next-generation cathode - better and chearper, pushing EVs beyond 500km driving range and soon to price parity with ICE (Internal Combustion Engine).
- LIB compostion <Fig.>:
NMC811 is a cathode compostion with 80% Ni 10% Mn, and 10% Co. NMC cthodes with different Ni-Mn-Co compositions have been around for almost 20 years. Following the initial commercil success of NMC111 (as NMC333, NMC cathodes have become mainstream, being used in BMW-i3, Chevy Bolt, or new Nissan Leaf.
- Industry has been improving NMC technology by steadily increasinng Ni content (e.g. NMC433, NMC532, or most recent NMC622)
- The cells have higher capacity and lower weight; the battery packs store more energy and better driving range.
- It is NCA (Ni80-Co15-Al), but are doped with Al as opposed to Mn.
- >Top Ni is largely responsible for cathode capacity, with Mn and Co helping chemical and structure stability.
- 20% increase of Ni content (from NMC622 to NMC 811) pushes the capacity of NMC811 to around 200 mAh/g (discharge potential 3.8V) <Fig.>.
- Co Price (LME):
Co is very expensive with very questionable supply chain (like Kawama mine, Congo). Note, cathode materials account for about 1/4 of the cell cost. While Ni and Mn prices are relatively low and steady, the cost of Co skyrockted by more than 200% (from $35K to $75K/ton in 2017 alone)
-
- NMC811 is more sensitive chemistry; requiring improved synthetic processes than NMC333 or even NMC622.
- NMC is synthesized with Ni in oxidised state $Ni^{3+}$, largely due to instabliity of $Ni^{3+}$ ion at high synthetic temperatures.
- NMC comes alog with undersirable residues mainly Li-based that need to be removed or pssivated.
- NMC is also sensitive to moisture and air.
- Fully oxidised $Ni^{4+}$ is reactive and so its exessive amount increase unwanted side reaction with the electrolyte.
- Capcity and cycle life don't necessarily go together for NMC811.
- Ni-rich NMC cathodes are sensitive to elevated temperature - release more $O_2$ than their counter parts; also undergo more structural changes. <Fig.>
- LG CHem and SK Innovation claim NMC811 will be in mass production by the end of 2019, but safety of NMC811suggest the timescals might be more conservative.
- NCA development:
- Note, NCA technology (by Panasonic and Tesla) is developing as well. It's very similar to NMC811, whether you consider its Ni-rich chemistry, superior capacity, more complicated manufacturing, or stability issues.
- Tesla supplied ESS (Energy Storage System) in South Australia.
- World's largest ESS with capacity 100/129MWh, which was constructed within 100 days.
- This ESS Farm having 315MW capacity is used as the backup of Neoen's Hornsdale Wind Farm near Jamestown to stabilize the grid in the summer..
- Tesla decided to use Samsung SDI cells to make it on time.
- The cost is estimated at some $50M.
|
- LIB Compostion: (Li/Ni/Co/Mn/Al)
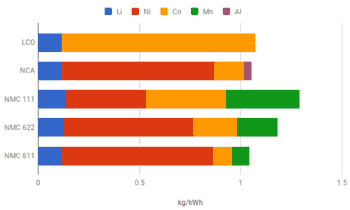
- LIB Capacity:
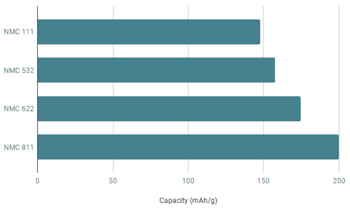
- LIB Temperature Sensitivity:
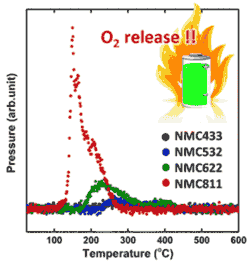
- LIB Structurel Changes:
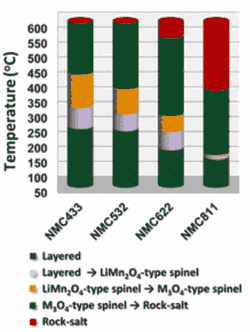
Gr7 |
Gr8 |
Gr9 |
Gr10 |
Gr11 |
Gr12 |
Gr13 |
|
|
|
|
|
|
Al |
Mn |
Fe |
Co |
Ni |
Cu |
Zn |
Ga |
Tc |
Ru |
Rh |
Pd |
Ag |
Cd |
In |
Re |
Os |
Ir |
Pt |
Au |
Hg |
Tl |
|