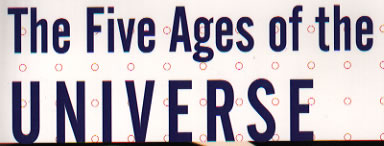
The Five Ages of the Universe
Cat: SCI
Pub: 1999
#: 0113b
Fred Adams and Greg Laughlin
01523u/18204r
Title
The Five ages of the Universe
宇宙の5段階
Author
Fred Adams & Greg Laughlin
F.アダムス、G.ローリン
Published
1999
1999年
Index
Tag
; ; 75% proton; Annihilation of dark matter; Billions years for intelligence; Black hole; Brown dwarf; Civilizations 1000; Cosmological decade; Dynamic relaxation; First three minutes; Four windows; Gravity or entropy; Heat death; Homogenous & isotropic; Horizon problem; ; More massive smaller; Neutron star; Proton decay; Relative isolation; Schwartzschild radius; Sun ranks 4th; ; ; Vacuum energy; White dwarf; ;
Why?
- This book gives us an answer to the primitive question since our
childhood: "Where are we from, and where are you going?"
- This is a biography of the universe; a detailed scientific inquiry
into the universe of the future, including the stars, galaxies,
etc.
- Biography needs a certain kind of bio-existence. It is also appealing
for us to imagine the future of life, though they may be totally
unlike us, but might be our descendant.
- この本には子供の頃からの基本的な疑問に対する一つの答えがある。それは「我々はどこから来て、どこへ行こうとしているのか?」という疑問である。
- これは宇宙の年代記であり、星や銀河を含む未来の宇宙に対する詳細な科学的な研究である。
- 年代記にはある種の生命の存在が必要である。また生命の未来を想像することは興味をそそるものである。たとえそれが我々と全く似ていなくとも。それらは我々の子孫かも知れないから...
Summary
要約
>Top 0. Introduction:
- Four windows to the universe:
Our biography of the universe plays out on four important size scales
planets, stars, galaxies, and the universe as a whole. Each of these
scales provides a different type of window to view the properties
and evolution of nature.
- Continued expansion:
The key question is whether the universe will continue to expand
forever or halt its expansion and recollapse at some future
time. Current astronomical data strongly suggest that the fate
of our universe lies in continued expansion.
- Structure of the universe:
Some aggregates of galaxies have enough mass to be bound together
by gravitational forces, and these galaxy clusters can be considered
as independent astrophysical objects in their own right. In
addition to belong to clusters, galaxies are loosely organized
into even larger structure that rather resemble filaments, sheets,
and walls. (the large scale structure of the universe)
- >Top Extreme emptiness:
It is difficult to comprehend the vast emptiness of our universe.
A typical galaxy fills only about on-millionth of the volume
of space that contains the galaxy, ant the galaxies themselves
are extremely tenuous. The universe is expanding but the galaxies
are not. Decoupled from the overall expansion of the universe,
the galaxies exit in relative isolation.
- Chances of stars:
Very little of the galactic volume is actually filled by the
stars, although galaxies contains billions of them. If you were
to drive a spaceship to a random point in our galaxy, the chances
of landing within a star are extremely small, about 10−22.
- Interstellar medium:
The space between the stars in not entirely empty. Our Milky
Way is permeated with gas of varying densities and temperatures.
The average density is only about one particle (one proton)
per cubic centimeter.
- Planet of other stars:
For the first time in history, planets in orbit about other
stars have been unambiguously detected. we now know with certainty
that planets are relatively commonplace in the galaxy, and not
jut the outcome of some rare or special event which occurred
in our solar system. Planets are important because they provide
the most likely environments for life to evolve.
- >Top Four forces of nature:
Nature can be described by four fundamental forces which
ultimately drive the dynamics of the entire universe; gravity, electromagnetic
force, strong nuclear force, and weak nuclear force.
- Gravity:
It is actually the weakest of the four. Since it has a long
range and is always attractive, gravity dominates the other
forces on sufficiently large size scales.
- Electromagnetic force:
On small size scales, in particular within atoms, the electromagnetic
force plays a vitally important role. It is ultimately responsible
for most of atomic and molecular structure. It is a whopping
1040 times stronger than the gravitational force. With only
gravity to bind an electron to a proton, a hydrogen atom would
be larger than the entire observable portion of our universe.
- Strong nuclear force:
It is responsible for holding atomic nuclei together. The protons
and the neutrons are held together in the nucleus by this force.
Without the strong force, atomic nuclei would explode in response
to the repulsive electric forces between the positively charged
protons. The range of it is about the size of a large atomic
nucleus, about 10−12 cm.
- Weal nuclear force:
This rather mysterious weak force mediates the decay of neutrons
into protons and electrons, and also plays a role in nuclear
fusion, radioactivity, and the production of the elements in
stars. A substantial fraction of the total mass of the universe
is most likely made up of weakly interacting particles. Such
particles tend to interact on very long time scales, they play
an increasingly important role as the universe slowly cranks
through its future history.
0.序:
- 宇宙を覗く4つの窓:
宇宙の伝記には、重要な4つの大きさなスケールが登場する。惑星、恒星、銀河、そして全体宇宙である。各々が自然の特性と進化を観察するための窓の役割を果たしている。
- 永遠の膨張:
宇宙は永遠に膨張し続けるのか。それともいずれは膨張が止まり再び崩壊していくのか。現在の天文学のデータによれば宇宙は膨張し続ける運命にあることを強く示唆している。
- 宇宙の構造:
いくつかの銀河の集合は、それらの質量によって、重力的に一まとめに結びつけられるており、これらの銀河団は独立した天体物理学的な物体とみなすことができる。またこうした銀河団として属しているだけでなく、さらに大きな構造にゆるやかにまとまって存在しており、それは繊維やシートや壁に似た構造に似ている。(宇宙の大組織構造)
- 極端な空虚:
広大に宇宙にはほとんど何もない。典型的な銀河は、その銀河を含む宇宙空間の体積のは百万分の一を占めているだけであり、その銀河自信も極端に希薄である。宇宙は膨張しているが、銀河は膨張しない。宇宙全体の膨張から取り残されて銀河は相対的に孤立するようになる。
- 恒星の割合:
銀河には数十億の恒星があるが、銀河の中身はほとんど空っぽである。もし宇宙船に乗って、ランダムに銀河の中に飛び出してみても恒星の漂着する確率は10−22である。
- 星間物質:
星間の空間は全く空という訳ではない。天の川には様々な密度と温度をもったガスで満たされている。その平均密度は1立方cmの中に粒子
(陽子) が1個である。
- 太陽系外惑星:
史上初めて、他の恒星の周りに軌道を描く惑星がはっきりと探知された。我々は今や確信をもって、惑星が銀河の中で比較的ありふれた存在で、太陽系だけに起きた希で特殊な出来事でないことを知っている。惑星が重要なのは生命が進化するのみ最適の環境を与えてくれるからである。
- 自然の4つの力:
自然には宇宙全体を動かす4つの基本的な力が存在している。重力、電磁気力、強い核力、弱い核力である。
- 重力:
実際には4つの中で最も弱い。但し、その到達力が長く、かつ引力だけなので、重力は十分に大きなスケールでは他の力を圧倒してしまう。
- 電磁気力:
これは小規模なスケール、特に原始の内部では重要な役割を果たす。これは大部分の原子および分子の構造の原因となる。これは重力の1040という途方もない強さがある。電子を陽子に結びつける力が重力だけとしたら、水素原子は宇宙の観測できる部分全体より大きくなってしまう。
- 強い核力:
これは原子核を結合させておく原因となっている。この力によって、陽子と中性子は原子核の中で結合されている。この強い核力がなければ、原子核は、正電荷をもつ陽子同士の斥力によって爆発してしまうだろう。この到達距離は大きめの原子核のサイズに近く、それは10−12 cmである。
- 弱い核力:
やや謎めいたこの弱い力は、中性子が陽子と電子に崩壊するのを媒介し、また核融合や放射能、星での元素の生成に役割を演じている。宇宙の総質量のかなりの部分は、弱く相互作用している粒子で作られているらしい。これらの粒子は非常に長い時間スケールで相互作用するので、宇宙が未来に向けてゆっくりと進むにつれてより重要な役割を演じるようになる。
- >Top War between gravity and entropy:
The great war between gravity and entropy determines the long-term
fate and evolution of astrophysical objects such as stars and galaxies.
Gravity pulls the star inwards, whereas the tendency for increasing
entropy favors dispersal of the stellar material. If gravity wins
this battle, the universe must eventually halt its expansion and
begin to recollapse some time in the future. On the other hand,
if gravity loses the battle, the universe will continue to expand
forever. Which one of these fates lies in our future path depend
on the total amount of mass and energy contained within the universe.
- >Top Cosmological decades:
We must take up the formidable challenge of establishing a time
line which depicts the universally interesting events that are likely
to transpire over the next 10100 years. The number 10100 is big;
attempting to visualize.
- The number of grains of sand in the world: 1023
- The number of stars in all the galaxies in our observable
universe: 1022
- The total number of protons in all galaxies: 1078
- τ=10η years
where ηis some number. The exponent η is the number of cosmological
decades.
- For example, the universe is currently only about 1010 years
or η=10 cosmological decades.
- One year after the big bang corresponds to 100 years, or
the zeroth cosmological decade. One-tenth or 10−1 year is thus
cosmological decade -1, and so on.
- The beginning of time corresponds to τ=0 when the big bang
itself took place; in terms of cosmological decades, the big
bang corresponds to negative infinity.
- Copernican Time Principle:
it states quire simply that the current cosmological epoch has no
special place in time. In other words, interesting things will continue
to happen as the universe evolves and changes. Although the available
levels of both energy and entropy production become increasingly
lower, this effect is compensated by the increasingly long time
scales available in the future.
- 重力とエントロピーの戦い:
重力とエントロピーとの大いなる戦いは、星や銀河という天体物理的な長期にわたる運命と進化を決定づける。重力は星を引き寄せる一方で、増加するエントロピーは恒星の物質の分散を助長する。もしこの戦いに重力が勝つと宇宙はいつか膨張をやめて、未来のある時に最収縮し始める。逆にもし重力がこの戦いに敗れると、宇宙は永遠に膨張する。この運命のどちらかが未来の道筋で待ち受けているかは、宇宙の中に含まれる質量とエネルギーの総量にかかっている。
- 宇宙年:
我々はこれから10100年に亘って起きそうな興味ある出来事を記述しなければならない。10100年は大きいが、それを視覚化すると
- 世界中の砂粒の数:1023
- 観測可能な宇宙にあるすべての銀河にある恒星の数:1022
- すべての銀河中にある陽子の数:1078
- τ=10η 年
ここでηはある数である。べき乗数のηを宇宙年とここで定義する。
- 例えば宇宙の現在の年齢は1010年、即ち10宇宙年という訳だ。
- ビッグバンの1年後は100年、即ち0宇宙年に相当する。その10分の1は10−1宇宙年となり、以下同様である。
- 時間の始まりは、ビッグバン自体が起きた時のτ=0に相当し、宇宙年でいえばマイナス無限大の宇宙年ということになる。
- コペルニクスの時間原理:
これは現在の宇宙時代は時間的に特別な時代ではないという考えである。言い換えればこれからも宇宙が進化し変化するにつれて興味深いことが起き続けるというものである。エネルギーとエントロピーの供給水準は、ますます低くなるが、その効果は未来に向かってだんだんと長くなる時間スケールによって埋め合わされる。
>Top 1. (-50<η<5):
The Primordial Era:
- First 10−35 seconds:
Imagine what it would be like to witness the beginning of time.
We would first notice that the universe is expanding and cooling
at a fantastic rate. During the first 10−35 seconds or so, the
universe expands so fast that adjacent point of space rush away
from each other at incomprehensible speeds. The size of a small
dot inflates to become large than the entire observable universe
of today.
- First microseconds:
In the extreme heat of the first microseconds, the temperature is
too hot for molecules, atom, and nuclei to be bound together. Even
protons and neutrons cannot exist. The universe is swarming with
mysterious elementary particles called quarks.
- The radiation field that was present at the beginning is still
with us today. It forms a sea of photons that fills all of space
and is called the cosmic background radiation.
- The quarks are made of both ordinary matter and antimatter,
with a slight excess of the former. For every 30 million antimatter
quarks, the universe contains 30 million and one quarks made of
matter. As the universe evolves and cools, the quarks and the
antiquarks annihilate with one another. Only the tiny excess fraction
of matter survives the process, eventually makes up all the matter
that we see in the universe today.
- >Top After 30 microseconds:
The leftover quarks begin to condense into protons and neutrons.
After about 30 microseconds, no free quarks are left.
- After 1 second:
Next, as the universe continue to cool, protons and neutrons begin
fusing into helium and other light nuclei. This process starts when
the universe is about one second old and ends rather abruptly after
a few minutes. The universe expands much too quickly for large nuclei
to com together.
- Inflation:
This period of superluminal expansion inflated the size of the
universe by an enormous factor, perhaps a 1030. After this short
but universe-altering epoch, the cosmos settle down into a state
of more ordinary expansion.
This
figure shows the size of the universe in both the standard big
bang theory and in the inflationary model. This smaller universe
could have been in causal contact with itself at some early time
in history and thereby produce the extreme uniformity that we
observe in our universe today.
- >Top Vacuum energy:
The uncertainty principle has important consequences for the concept
of a vacuum. The vacuum cannot really be empty. This apparently
empty space is filled with particles flickering briefly in and out
of existence. The energy required to make these particles is borrowed
form the vacuum and then quickly repaid when the particles annihilate
each other and subsequently disappear back into nothingness. These
particle are called virtual particles. They live on borrowed
time and always annihilate just after their spontaneous appearance
out of the vacuum.
- Negative pressure:
As Einstein's famous formula E=m×c2 illustrates, energy is equivalent
to mass, and these large vacuum energies must have correspondingly
large gravitational effects. The vacuum energy, however, has the
curious property of exhibiting a negative pressure. This negative
pressure is larger than the mass energy and hence the press effects
dominate the expansion.
1.(-50<η<5) 原始時代:
- 最初の 10−35 秒:
時の始まりを目撃する場面を想像してみよう。宇宙がすばらしい速さで膨張しつつ冷えていくことに気づくだろう。最初の10−35 乗位の間に、宇宙は急激に膨張し、隣り合う空間の部分が想像を絶する速度でお互いに離れていく。小さな点に過ぎなかった宇宙が膨張して現在観測できる宇宙全体より大きくなる。
- 最初のマイクロ秒:
最初のマイクロ秒の極端な熱の中では、分子や原子や原子核がまとまっているには高温すぎる。陽子や中性子でさえも存在できない。宇宙はクォークと呼ばれる不思議な素粒子で一杯だった。
- 宇宙の始まり頃に存在していた放射は、今でも残存している。それは宇宙空間のすべてを満たす光子の海を形成し宇宙背景放射と呼ばれている。その有効温度は絶対温度2.7度まで下がってしまった。
- クォークは通常の物質と反物質の両方で作られているが、宇宙には3千万個に1個の割合で物質のクォークの方が反物質のクォークより多く存在していた。宇宙が進化し冷えると、クォークと反クォークは衝突して消滅する。ほんの少し多い物質だけが消滅を免れて生き残り、結果的にすべての物質を作っている。
- 30マイクロ秒後:
宇宙誕生後30ミリ秒経つと、自由クォークは残されていない。
- 1秒後:
次に宇宙が引き続き冷えるに従って、陽子と中性子はヘリウムなど軽い原子核を融合し始める。このプロセスは宇宙誕生後ほぼ1秒後にスタートし、数分後に突然終了する。宇宙は、大きな原子核がまとまるには膨張が速すぎる。
- インフレーション:
超高速の宇宙膨張の器官に宇宙の大きさはおそらく1030の倍率に膨張した。この短い間の宇宙そのものを変えてしまう期間の後、宇宙は通常の膨張状態に落ち着いた。
←左図は、標準的なビッグパン理論と、インフレーション理論での宇宙の大きさを示す。宇宙が小さければ、ある早い時期に全体が因果的につながっていても不思議ではなく、その結果、現在観測されるような極端に一様な宇宙を作り出した。
- 真空エネルギー:
不確定性原理は、真空の概念について重要な結論をもっている。真空は真の真空ではあり得ない。不確定性原理からすれば、この空っぽの空間も、生成と消滅を繰り返す仮想的な粒子で充満している。このような粒子を作るのに必要なエネルギーは真空から一時的に借用し、その後粒子同士の衝突で消滅して無に帰ると、すばやく返済される。これらの粒子は仮想粒子と呼ばれる。仮想粒子は、借りた時間上で存在し続け、真空から自発的に生まれた直後に消滅する。
- 負の圧力:
アインシュタインの有名なE=m×c2式が示すようにエネルギーは質量と等しい。それゆえ真空の大きなエネルギーは大きな重力効果をもっているはずである。しかし真空エネルギーは負の圧力という奇妙な特性をもっている。この負の圧力は質量エネルギーより大きいので、その圧力効果が膨張を左右することになる。
- >Top Horizon problem:
One important property of our universe is that it look the same
in all directions. In particular, the temperature of the cosmic
background radiation is nearly identical in different directions
in the sky. And this communication must have taken place before
the universe was 300,000 years old, when this radiation last interacted.
Without inflation, such regions are not able to communicate each
other and the universe suffers from the horizon problem.
- The background radiation was last able
to interact with matter during 300,000 years old and the background
photons we see today have been streaming freely since that time.
These region have expanded to a size of about 300 million light-years
at the present epoch. However we are sampling regions separated
by the size of the entire observable universe today, distances
larger than 20 billion light-years, yet the observed temperatures
of the cosmic background radiation are virtually identical,
the same to a few parts in 100,000. There is no easy why the
temperature of regions which were completely out of contact
should be so uniform. The dilemma constitutes the horizon problem.
- >Top Expanding universe:
The expansion and evolution of the universe is described by Einstein's
theory of general relativity, which incorporated gravity into a
fundamental description of space-time. Einstein went so far as to
unnecessarily complicate his theory to allow for a static universe.
After the expansion was discovered, however, cosmologists quickly
understood that the original, unmodified equations provided the
best description of our continually growing universe.
- Cosmic background radiation:
The entire universe is pervaded by a background sea of radiation.
As the universe expands and cools, the radiation is stretched out
to lower energy and eventually ceases to interact.
- How do we know that this faint background of microwaves is
actually a fossil signature of the big bang?
- When equilibrium is attained, the spectrum of the radiation,
the amount of energy emitted at each wavelength, approaches
a particular form known as a black body.
- >Top Another property of the observed background radiation was
imprinted in the blazing past of the early universe.
(cosmological principle: universe is homogeneous
and isotropic.)
- 地平線問題:
我々の宇宙の重要な特性の一つは、あるゆる方向に同じように見えることである。特に問題なのは、宇宙背景放射の温度が異なる方向でほとんど同じことである。このコミュニケーションは、放射が最後に違いに関係していた宇宙誕生後30万年以内に起きたはずである。インフレーションがなければ、このような領域は互いに連絡がとれず、宇宙は地平線問題を抱えることになる。
- 背景放射は、30万年後までの時期に、最後に物質と相互作用して以来、今見られる背景の光子は自由に跳び続けてきた。この領域は今や3億光年にまで広がった。しかしながら我々は、観測可能な宇宙全体の大きさ、つまり200億光年以上の距離を隔てた領域をサンプリングしている。にもかかわらず宇宙背景放射の観測された温度は実質的に全く同じで、誤差は10万分の1しかない。なぜ全く接触の範囲外にある領域の温度が極めて一様なのかの理由は簡単ではない。このジレンマが地平線問題である。
- 膨張する宇宙:
宇宙の膨張と進化は、時空の基本的な重力を取り入れたアインシュアインの一般相対性理論によって記述される。アインシュタインは、静的な宇宙を認めるために敢えて自分の理論を複雑に修正してしまった。後で宇宙の膨張が発見されるや否や、宇宙論学者たちは、当初の修正前の方程式によって、我々の成長する宇宙が最もうまく説明できることに気づいた。
- 宇宙背景放射:
宇宙全体に放射の背景の海が広がっている。宇宙が膨張し冷えるにつれて放射はより低いエネルギーにまで引き延ばされ 、ついには粒子との相互作用をやめてしまう。
- この微弱なマイクロ波背景がビッグバンの化石的な痕跡であることがなぜわかるのか。
- 平衡状態に達すると放射のスペクトル即ち各波長で放出されるエネルギー両は、黒体と呼ばれる特殊な形態に近づく。
- もう一つの特質は、初期の宇宙の炎につつまれた過去に刻印されたものである。(宇宙原理:宇宙が一様で等方であること)
>Top Quark and Antiquarks:
- During the first microsecond of cosmic history, the matter content
of the universe lived in the form of quarks and their antimatter
counterparts called antiquarks.
- In rough terms, our universe contains about 1078 protons
and neutrons, with a relatively negligible admixture of antiprotons
and other antimatter particles. Both matter and antimatter are
fundamentally on an equal footing.
- Back in the earliest moments of cosmic history, physical processes
that do not conserve baryon (like protons and neutrons) number
began to operate. The ensuing microscopic reactions produce
a net excess of quarks in some regions of the universe.
- The reactions must be able to sense or follow the direction
of time, which is defined by the expanding universe. Thus, in
order to generate a net excess of matter, the universe must
provide reactions that violate baryon number, take place out
of equilibrium, and are not time reversible.
- This change of phase occurs as the universe falls through
a temperature of one trillion degrees kelvin and attains the
density of nuclear matter, one quadrillion times the density
of water. With these background conditions, protons and neutrons
burst into existence.
- >Top The first 3 minutes:
About one second after the big bang, the background temperature
dropped to 10 billion degrees K. With a density 200,000, the universe
was cool enough to begin fusing protons and neutrons into light
atomic nuclei. A great deal of helium was synthesized, with smaller
admixtures of deuterium and lithium. This nuclear activity continued
for about 3 minutes. At this juncture, the temperature fell to one
billion degrees K and the density to only 20 times the density of
water. Nuclear reactions then abruptly shut down.
- Most of the universe, about 75% by mass, emerged unprocessed
in the form of protons (hydrogen).
- If nucleosynthesis in the early universe had continued indefinitely,
all of the protons and neutrons would have eventually fused
into iron.
- >Top Dark Matter:
The total amount of stellar mass in the universe appears to be surprisingly
small, however, less than 1% of the closure density. In light of
stellar observations and the theory of nucleosynthesis, the universe
must contain 2 to 8 times more mass in baryons than is accounted
for in ordinary stars.
- Astronomers measure the speed at which stars are orbiting
about the centers of other galaxies. The larger the observed
orbital speed, the more mass required. This accounting procedure
implies that most of the mass contained in galaxies resides
in the outer galactic halos; perhaps 100 times more mass than
the stars themselves. The total mass of galactic halos thus
accounts for about 10% of the closure density.
- Observations also determine the amount of mass distributed
over regions the size of galaxy clusters. In this case, we measure
how fast the galaxies themselves are orbiting about the cluster
centers. Although somewhat uncertain, these measurements indicate
that galaxy clusters contain roughly 30% of the mass required
for closure. This large reservoir of material is 4 to 15 times
more massive than the total amount of baryonic matter; substantial
fraction of the matter in the universe must reside in nonbaryonic
form.
- Two different dark matters:
The first regime includes particle with masses of about 10 to
100 times the mass of a proton. Such heavy particles are relatively
slow moving and are generally known as cold dark matter.
- The second possible regime includes lighter particles, with
approximately a billion times less mass. These light particles
have relativist speeds and are known as hot dark matter.
クォークと反クォーク:
- 宇宙の歴史の最初の数マイクロ秒の間に、宇宙の物質はクォークだけでなく、反クォークと呼ばれる反物質の相手も存在していた。
- 我々の宇宙には、1078の陽子と中性子があり、ほとんど無視できる分量の反陽子と他の反物質素粒子がほんの少しだけ混ざっている。物質と反物質とはどちらも基本的には対等の資格をもっている。
- 宇宙誕生から1マイクロ秒経つずっと前 (陽子や中性子のような) バリオン数を保存しない物理プロセスが働いていた。続いて起きたミクロの世界の反応が宇宙のある領域でクォークの過剰をもたらした。
- 反応は、膨張する宇宙によって決まる時間の方向を感知し、それに沿ったものでなければならない。このように正味の質量増加を生じさせるためには、バリオン数を破り、平衡状態から離れた反応が起き、時間逆転できないように進むことが必要である。
- 宇宙の温度が1兆度に下がり、水の密度の1015倍にあたる核物質の密度になる時に相変化が起こる。こうした背景の条件で陽子と中性子が突然存在し始める。
- 最初の3分間:
ビッグバンの約1秒後、背景温度が100億度Kまで下がりいる。密度は200,000で、宇宙は十分に冷えて、陽子と中性子が軽い原子核へと融合する。膨大なヘリウムと、少量の重水素やリチウムの混合物と共に合成される。この核反応は約3分間続く。この時、温度は10億Kまで下がり、密度は水の20倍まで下がっている。そして核反応は突然止まる。
- 宇宙の大部分、質量で約75%は、陽子(水素) のまま反応しないで残る。
- もし初期宇宙の元素合成が限りなく続いていたら、陽子と中性子のすべては最終的に融合して鉄になっていただろう。
- 暗黒物質:
宇宙における星の総力は驚くほど小さいようで、宇宙が閉じる密度の1%以下であろう。星の観測結果と元素合成の理論によって、宇宙には通常の星の2ー8倍のバリオンの質量を含んでいる。
- 天文学者は他の銀河を周回している星の速度を測定する。周回速度が大きいほどより多くの質量が必要になる。この計算によって銀河に含まれる質量の大部分は外側の銀河ハローに存在しており、おそらく星の100倍もの質量がある。このように銀河ハローの全質量は、宇宙が閉じる質量の約10%を占める。
- また観測結果から銀河団規模の領域に分布している質量を推定できる。我々は、銀河がどの位の速さで、その銀河団の中心を回っているかを測定する。多少不確実だが、銀河団は宇宙が閉じるのに必要な質量の約30%を含んでいる。この貯蔵量は、バリオン物質総量の4〜15倍も多い。このことは宇宙物質のかなりの部分が非バリオンの形態で存在していることを意味している。
- 2種類の暗黒物質:
最初のグループには、陽子の質量の10〜100倍の質量をもつ粒子が含まれる。これらの重い粒子は、相対的にゆっくる動いており、冷たい暗黒物質と呼ばれている。
- 二番目のグループにはおよそ10億分の1の質量の軽い粒子が含まれる。これらの軽い粒子は相対論的速度をもっており、熱い暗黒物質と呼ばれている。
>Top2. (6<η<14):
The Stelliferous Era
- Galaxy formation:
After the fireworks of the first 3 minutes, for the next 300,000
years, space was filled with a nearly featureless sea of hydrogen
and helium nuclei, photons, and free electrons, all in a state of
constant interaction known as thermal equilibrium.
- When the universe cooled down to a temperature of about 3000K,
electrons and atomic nuclei combines to form ordinary atoms,
mostly hydrogen. As the radiation temperature plummets, the
photons suddenly lack the energy required to separate electrons
from the nuclei, and the particles combine to form neutral atoms.
Then newly complete hydrogen and helium atoms are then free
to collapse under the influence of gravity. The ensuing collapse
produces vast aggregations of stars, gas, and galaxies.
- >Top Star formation:
The first stars were born at about the same time as the first galaxies.
At the present epoch, stars from within molecular clouds, vast aggregations
of molecular gas residing in galactic disks. These clouds, which
often contain the mass of a million Suns, are much denser and colder
than the surrounding interstellar gas. Stars are born out of the
collapse of molecular cloud cores, small subcondensations which
are scattered throughout the much larger volume of a cloud.
- These core regions are threaded by magnetic fields, which
provide a vital source of pressure to help support the cores
against gravitational collapse. The magnetic fields gradually
diffuse outward and the central regions grow increasingly concentrated.
Soon after the inevitable catastrophe of collapse begins, a
small pressure-supported protostar appears at the very center of the collapse flow.
- These cores spin extremely slowly, about one rotation per
million years. This slow rotation imbues the system with a substantial
amount of angular momentum. As a result, not all of the mass
falls directly onto the nascent star. This nebular disk of gas
and dust provides a friendly environment for making planets.
- During the main collapse phase, the central protostar and
its nebular disk are surrounded by an inward flow of gas and
dust. This infalling envelope is thick enough to largely obscure
an outside view of the forming star. The original visible radiation
emitted by the central star is reprocessed so that forming stars
can on be observed at infrared wavelength. For this reason,
bona fide forming stars were not unambiguously identified until
the 1980s.
- At the beginning, stars derive most of their energy from gravitational
contraction. As the star shrinks, its central core heats up,
and hydrogen fusion is eventually initiated. Once sustained
nuclear fusion reactions finally begin, the star is fully formed.
- >Top Right here right now:
Imagine shrinking Sun down to the size of a grain of sand. Earth
and its orbit traces a circle about an inch in diameter. The distance
to Pluto shrinks to 2 ft. At this scale, the nearest star system
(Alpha Centauri) is 2 miles away. One can hardly overemphasize the
relentless emptiness of our present-day galaxy. And the galaxy is
a million times denser than the universe as a whole.
- Cast of stellar characters:
To sustain nuclear reactions in their interiors, gaseous celestial
bodies must contain at least 8% of the solar mass. The Stelliferous
era is rife with failed stars (brown dwarfs) that are too small
to generate nuclear power. Brown dwarfs effectively store away unprocessed
hydrogen fuel. When the Degenerate Era arrives, this investment
finally pays off.
- Stars live in galaxies which have masses of about 1011 solar
masses, and stars are made of hydrogen atoms of about 10−24
grams or about 10−57 solar masses.
- >Top Since the stars visible to the naked eye are almost exclusively
more massive than the Sun, one might think that the Sun is a
rather small star. This conjecture is simply not true. Of the
50 nearest known stars, the Sun ranks a very respectable 4th and is thus relatively large. The lower mass stars, mostly red
dwarfs with less than half the mass of our Sun, completely dominate
the stellar population by number.
2.(6<η<14):
星多き時代
- 銀河の誕生:
始めの3分間の後、30万年の間、宇宙空間は水素、ヘリウムの原子核、光子、自由電子が熱平衡状態で常に相互作用をしている特徴のない海で満たされていた。
- 宇宙が約3000度Kまで下がった時、電子と原子核が結合し、通常の原子を形作る。そのほとんどは水素である。放射の温度が急に下がると、光子は電子を原子核から分離するのに必要なエネルギーを持たなくなり、粒子同士が結合して中性の原子が生まれる。新しくできた水素とヘリウムの原子は重力の影響で自由に合体するようになる。銀河の誕生である。沢山の粒子がくっつくと、星やガスや銀河が生まれる。
- 星の誕生:
最初の星は、最初の銀河とほぼ同じ時期に誕生した。現在は、星は銀河円盤中にある分子ガスの広大な集まりである分子雲の中で作られる。この雲はしばしば太陽の百万倍の質量があり、周囲の星間ガスよりも遙かに高密度で冷たい。星は分子雲の中心核が潰れることによって生まれ、小さな準凝縮体が、その雲の遙か大きな体積を占めて分散している。
- この中心核の領域は磁場によって織りなされており、それによって中心核が重力崩壊を防ぐ圧力になっている。磁場は徐々に外部に消散し、中心領域はどんどん凝縮していく。やがて避けられない崩壊のカタストロフィーが始まるとすぐに、圧力に支えられた小さな原始星が、崩壊流の中心に現れる。
- 中心核は、数百万年に1回転位のゆっくりした速度で回転している。この遅い回転によって、かなりの角運動量が系に行き渡る。その結果、すべての質量が発生しようとする星に直接落下することはない。このガスと塵の星雲状円盤が惑星を作るのに好環境になる。
- 本格的な崩壊段階になると、中心の原始星と星雲状円盤は、ガスと塵の内側への流れによって囲まれる。この落下するエンべロープは厚いので、形成されつつある星を外からは見えなくなる。中央の星からの本来の可視放射は再処理され、形成されつつある星は赤外線の波長によって観察できる。この理由で、形成されつつある星は1980年代までははっきりと識別されなかった。
- 誕生直後、星はエネルギーのほとんどを重力収縮からもらっている。星が収縮すると、その中心核は加熱し、やがて水素の融合がはじまる。一旦、継続する核融合反応が始まると、星はようやく一人前になる。
- 現時点の位置関係:
太陽を砂粒の大きさに縮小してみよう。地球とその軌道は直径2.5cmの円を描くことになる。冥王星までの距離は60cmとなる。このスケールでは、最も近い星のケンタウルスα星までは4km弱離れた所にある。現在の銀河のこの容赦ない空虚さは強調しすぎることはないほどである。しかもそのスカスカの銀河は宇宙全体より百万倍以上も密度が高いのである。
- 恒星の役者たち:
内部での核反応を維持するためにはガス状の天体は少なくとも太陽質量の8%をもつ必要がある。「星多き時代」は、褐色矮星と呼ばれる出来損ないの星だらけである。褐色矮星は水素燃料を効率よくため込んでいる。「縮退する時代」がくるとこの投資が最終的には役にたつ。
- 星は太陽質量の約1011の質量を持った銀河の中に存在する。そして星は、約10−24 gで、太陽質量の10−57倍に相当する水素元素からできている。
- 肉眼で見える星々はほとんどが太陽より重いので、太陽はどちらかというと小さな星だと考えるに違いない。この推測は正しくない。我々の近くにある50個の星の中で、太陽はいみじくも4番目にランクされており、比較的大きいのである。低質量の星はほとんどが赤色矮星だが、星の数としては圧倒的に多い。
- >Top The fate of the Sun:
- Radioactive dating tells us that the oldest meteorites were formed
4.5 billion years ago. After the genesis of our solar system, the
Sun contracted over the next few millions years until its core became
hot enough for hydrogen fusion to take place. These nuclear reactions
have thus been powering the Sun for the last 4.5 billion years.
At the center of the Sun, the temperature is 16 million degrees
K. So far, about half of the hydrogen at the center has been burned
into helium. Enough hydrogen remains in the extended core to keep
the Sun shining for another 6 billion years. Nevertheless, in 6
billion years, the Sun will be about twice as bright as it is today.
- The brighter and hotter Sun presents grave
consequences for life on Earth. The greenhouse effect can develop
into a vicious cycle. Warming oceans release carbon dioxide
and water vapor, which lead to further heating which in turn
released even more gas into the atmosphere. As this runaway
greenhouse effect gather momentum, the oceans evaporate entirely
and scald Earth with a sterilizing atmosphere. Within a few
billion years, our world now green and flowering with life will
closely resemble the present-day Venus, with a hellish atmosphere
fueled by a runaway greenhouse effect.
- After the hydrogen in the solar core has been entirely converted
to helium, a shell of material just above the
core continues the fusion process. Because the central temperature
is too cool to fuse helium into heavier elements, the core lacks
an energy source and cannot support the weight of the overlying
bulk of the Sun. As the core shrinks, the temperature of the
central region increased accordingly. The spent helium core
continue to shrink, and the power output of the Sun steadily
rises. Paradoxically, as the Sun becomes more luminous, its
surface grow cooler (from 6,000K to 3,000K) This surface cooling
comes about because the excess energy produced near the core
is partially deposited in the middle layers of the Sun. The
Sun evolves to become brighter, large, redder, and cooler than
the yellow orb we see today.
- A billion years or so after hydrogen is first
depleted in the center of the Sun, the exhausted central core
becomes so dense that most of the pressure is provide by degenerate
electrons. A stellar object supported by this degeneracy pressure
is called a white dwarf and is about the radial size of Earth.
An expanding red giant basically consists of a central white
dwarf surrounded by an extremely
deep and diffuse stellar atmosphere. The central core reaches
about one million times denser than water. Two curious properties
of degenerate material are:
- More mass is piled onto the white dwarf, it radius steadily
shrinks.
- When degenerate matter is heated, an increase in temperature
produces neither expansion nor an increase in pressure.
- The core of a red giant is rapidly transformed into a colossal
helium bomb. This tremendous ensuing burst of energy, known
as the helium flash, is so powerful that it heaves the dense
core out of degeneracy and into a more expanded and stable configuration.
At the peak of its red giant phase, the Sun will be 2,000 times
brighter than it is now.
- 太陽の運命:
- 放射性年代測定によれば、最古の引責は45億年前に作られた。太陽系が生まれた後、太陽は数百万年をかけて、その中心核が水素融合を起こすのに十分なほど熱くなるまで縮小した。この45億年の間、核反応がこうして太陽にパワーを与えてきた。太陽の中心では、温度は1600万度Kにもなる。すでに約半分の水素が燃焼してヘリウムになった。中心核の周辺には太陽がさらに60億年の間照らし続けるのに十分な水素が残っている。
- より明るく熱い太陽は、地球上の生命に重大な影響を与える。温室効果が悪循環を引き起こす。温暖化した海洋は二酸化炭素と水蒸気を放出し、それがさらに加熱を進め、これまでにない多くのガスが大気中に放出される。手に負えなくなった温室効果が勢いを増すにつれて、海洋は完全に蒸発し、地球は灼熱の大気で焦がされて不毛の地となる。数十億年以内に緑と命の花が咲く我々の地球は、手に負えない温室効果で煽られた地獄のような大気をもつ現在の金星によく似た姿になるだろう。
- 太陽の中心核の水素がすべてヘリウムに転換された後、中心核のすぐ上の物質の殻が融合を続ける。中心温度がヘリウムを融合させて重い元素を作るには中心の温度が低すぎるので、中心核はエネルギー源が不足となり、かさばった太陽の容積の重さを支えきれなくなる。中心核が縮小すると、それに応じて中心領域の温度は上昇する。ヘリウムの中心核はさらに縮小を続け、太陽のパワーは着実に増加する。矛盾するようだが、太陽の輝きが増すにつれてその表面温度は冷えてくる。(6000度Kから3000度Kへ)この表面の冷却は中心核付近で作られる過剰なエネルギーが一部、太陽の中間層に蓄積するからである。太陽は進化して、今我々が見ている黄色い天球より明るく大きく赤くそして冷たくなる。
- 太陽の中心で水素が枯渇してから10億年も経つと、使い尽くされた中心核は非常な高密度になり、圧力の大部分は縮退した電子によって供給される。この縮退圧によって支えられた天体は白色矮星と呼ばれ、地球の半径ほどの大きさである。膨張していく赤色巨星は、中心にある白色矮星の周囲に極度に厚い星の大気が拡がったものである。中心核は、密度は水の100万倍に達する。縮退物質には2つの奇妙な特質がある。
- 白色矮星にさらに質量を詰め込むと、その半径は着実に縮小する。
- 縮退物質を加熱して温度が上がっても、膨張もせず圧力も上がらない。
- 赤色巨星の中心核は、急速に巨大なヘリウム爆弾に変身する。赤色巨星のエネルギー生成率は、銀河のすべての星が生むパワーにも匹敵する。ヘリウムフラッシュとよばれる猛烈なエネルギーの噴出は非常に強力で、高密度の中心核を縮退から解き放ってもっと膨張した安定な形態に移行させる。赤色巨星のピーク時には、太陽は今より2,000倍も明るくなるだろう。
- >Top Lost in space:
- As the Sun churns through its life cycle, Earth's biosphere is scheduled
to be completely destroyed by a runaway greenhouse effect in about
2 billion years.
- Computer simulation shows Earth, the Moon, and the Sun reacting
to a visit from a red dwarf with 1/4 of a solar mass. The simulation
predicts that Earth would be ejected from the solar system at
high speed. However, the chances of such an event taking place
before the Sun turns into a red giant are slim: 1 in 10^5.
- With this speed, earth crosses the orbit of Pluto within a
few years and leaves the solar system. Soon, the entire planet
is plunged into a permanent deep freeze,. At 77 degrees K, the
nitrogen falls as rain onto the frigid snowy surface. The oxygen
from the atmosphere also rains out of the frozen skies. Geological
activity is driven by heat from the decay of radioactive elements
and lasts for billions of years, longer than the remaining lifetime
of the Sun.
- The fate of massive stars:
The nature of stellar death depends on stellar mass. Single stars
that contain more than half of solar-mass, but less than 8 times
are destined to share the same fate. At the end of their lives,
they disgorge huge quantities of hot gases (called planetary nebulae)
and leave behind white dwarfs composed primarily of carbon and oxygen.
- 15-solar-mass star:
The stellar lifetime is very short; the star burns its central
stores of hydrogen into helium within only 10 million years.
The temperature in this core is more than 100 million degrees
and the density is about 1,000 times that of water.
- One reason why stars spend most of their lives in the hydrogen-burning
phase is because the fusion of hydrogen into helium is the most
exothermic nuclear reaction.
- After carbon burning has run its course, the structure of
a massive evolving star bears a vague resemblance to an onion.
- >Top The diagram of a supernova:
This diagram shows the schematic structure
of a very evolved star of high mass, just a few minutes before a
supernova explosion.
Each 'onion skin' represents a layer of nuclear burning near the
central inert iron core. Only the central region of the star is
shown here, the rest of the star consists of a thick envelope of
unburned hydrogen.
- The fusion of iron into even heavier elements (silver or gold)
does not lead to the release of energy, but rather requires
the absorption of energy.
- When the core density approaches 1014 grams per cm3, free
electrons and protons combine to produce neutrons and neutrinos.
This thick soup of neutrons resembles a single gigantic nucleus.
- >Top Supernova:
The collapse of an iron core in a massive star, followed by
the bounce at nuclear densities and the ensuing shock wave,
is called a supernova. The shock wave
is strong enough to completely blow apart the outer layers of
the star. Heavy elements (including gold, lead, and uranium)
are synthesized within the shock itself. At the center of the
supernova explosion, the dense core of neutrons can remain behind
as a neutron star.
- Alternatively, of the core is heavier than a few times the
solar mass, it may collapse into a black hole.
If a sufficiently massive star explodes violently and is efficient
at expelling stellar material, then so stellar remnant of any
kind is left behind.
- Supernovae are the most dramatic phenomena in stellar evolution.
For a single second, the amount of energy produced by a supernova
rivals the total energy emitted by all of the stars within the
visible universe. For days the residual explosion shines as
brightly as the entire galaxy that the dying star called home.
- >Top The fate of low-mass stars:
- In Johannesburg in 1916 an astronomer reported an unremarkable
star, far too faint to be seen with the naked eye, attracted
his attention because it was slowly changing its position; Proxima
Centauri, just one out of billion of red dwarfs in our galaxy.
- Proxima has about 15% of Sun's mass, an density several time
that of lead, and a power rating 400 dimmer than our Sun. Proxima
must resort to convection, a process in which the turbulent
motion of stellar gas physically carries energy away from the
center.
- Complete convention, couple with an underwhelming power output,
allows red dwarfs to survive almost unaltered, long after the
higher mass stars have turned into white dwarfs or self-destructed
in supernova explosions.
- 宇宙の迷子:
- 太陽が激動のライフサイクルを進むにつれて、地球上の生物圏は、ほぼ20億年の内に、手がつけられない温室効果によって完全に破壊される運命にある。
- コンピュータ・シミュレーションによれば、太陽質量の1/4の質量の赤色矮星の来訪による地球と月と太陽の反応を示す。地球は高速で太陽系から投げ出されるだろうことを予測している。しかしこのような事象が、太陽が赤色巨星になる前に起こる確率は、約10万分の1に過ぎない。
- このスピードだと地球は数年以内に冥王星の軌道を横切って太陽系を飛び出してしまう。まもなく惑星全体は永久凍結の状態に入る。77度Kでは窒素が雨となって極寒の雪に覆われた地表に降り注ぐ。大気中の酸素もまた凍りついた空から雨となって降る。地質学的な活動は放射性元素の崩壊からの熱によって行われており、太陽の残り寿命より長く数十億年に亘って持続する。
- 重い星の運命:
星の死の様子は星の質量に依存する。太陽の質量の半分以上で8倍以下の単独の星の場合は同じ運命をたどることになる。このような星はその生涯の終わりに、膨大な量の熱いガスをはき出し (惑星星雲と呼ばれる) おもに炭素と酸素からなる白色矮星を後に残す。
- 15倍の太陽質量の星:
その星の生涯は非常に短い。星は1,000万年以内の中心部の水素を燃やしてヘリウムにする。この中心部の温度は1億度Kであり、密度は水の1,000倍となる。
- なぜ星はその寿命の大半を水素燃焼の局面で過ごすのかの理由は、水素からヘリウムへの融合がもっとも発熱の激しい核反応だからである。
- 炭素の燃焼プロセスのあとに、質量の大きな星の構造はタマネギにどことなく似てくる。
- 超新星の断面:
左図は、超新星爆発の数分前の高質量の非常に進化した星の断面を示している。それぞれのタマネギの皮は、不活性な鉄の中心核近辺の核燃焼の層を示している。ここには星の中心領域だけを示している。星の残りの部分は燃焼していない水素が厚く包んでいる。
- 鉄がさらに重たい元素(銀や金など)に融合するにはエネルギーの放出にはならず、むしろエネルギーの吸収が必要になる。
- 中心核の密度が1014 g/cm3に近づくと自由電子と陽子は融合して中性子とニュートリノを作り出す。この中性子の厚いスープは一個の巨大な原子核のようになる。
- 超新星:
重たい星の鉄の中心核が潰れて原子核の密度になると跳ね返って、外部に衝撃波ができるのを超新星と呼んでいる。衝撃波は非常に強いので星の外側の層を完全に吹き飛ばしてしまう。重たい元素(金、鉛、ウランなど)はこの衝撃波の中で合成される。超新星爆発の中心では高密度の中性子が残り中性子星となる。
- もし中心核は太陽質量より数倍も重い場合には、それは潰れてブラックホールになる。もし十分重たい星が激しく爆発し星の物質を効率よく吹き飛ばしてしまう場合には、星の残骸が後に何も残らない。
- 超新星は星の進化の中で最も劇的な出来事である。ほんの1秒の間、超新星によって放出されるエネルギーは見える範囲の宇宙にあるすべての星から放出さる全エネルギーに相当する。爆発の残骸は、何日もの間死にゆく星が棲んでいた銀河全体と同じくらいの明るさで輝く。
- 軽い星の運命:
- 1916年ヨハネスブルグで、一人の天文学者が目立たない星の発見を報告した。それは肉眼で見るには光が弱すぎるが、ゆっくりとその位置を変えていることが興味を引いたのである。それがケンタウルス座プロキシマであり、我々の銀河にある何十億となる赤色矮星の一つであった。
- プロキシマは、太陽質量の15%しかなく、鉛の数倍の密度ももち、エネルギー出力は太陽の1/400である。プロキシマ星は対流に頼っており、星のガスの乱流運動によって物理的にエネルギーを中心から運び出している。
- 完全な対流は、その圧倒的に小さなエネルギー出力と相まって、赤色矮星をほとんど姿を変えずに生き延びさせ、質量の大きな星が白色矮星や超新星爆発で自滅した後もずっと長く生き続ける。
- >Top Extraterrestrial life:
- A planet orbiting a relatively massive star - such as Deneb in Cygnus
- is not likely to harbor an alien civilization. A 10-solar mass
star lives for only 10 million years before destroying itself in
a supernova.
- Two lines of evidence suggest that a long time is require
for advanced civilizations to arise. In the one example we know,
the evolution of intelligence required billions of years.
- We have no indications that any other civilizations exist.
In particular, no extraterrestrial societies have contacted
us. If technological civilizations could easily arise in short
periods of time, then we might expect some relatively nearby
star to broadcast detectable signals.
- >Top With moderate confidence, we estimate that the galaxy contains
roughly one billion habitable planets.This history of life on
Earth suggests that once the evolution of life begins, the development
of complexity, intelligence, and even technology is reasonably
likely. We suggest that roughly one in ten thousand of the habitable
planet develop life. Assuming that civilizations with technological
capabilities do not readily destroy themselves, we estimate
that the total number of civilizations in the galaxy is roughly 1,000.
- The isolation imposed by the enormous distances between stars
is vast and profound. Suppose that the nearest intelligent civilization
is 3,000 light-years away. For comparison, our current census
of nearby stars is complete out to a distance of only 15 light-years.
- Although a thousand civilizations could conceivably live within
our galaxy, a great deal of luck is necessary to establish contact.
- Colonization of the galaxy:
To estimate the time required to travel the length of the galaxy
by a random walk, we can assume that the galaxy is 30,000 light-years
across, and the step length travel by a life-carrying meteor is
a few light-years, the typical distance between stars. The meteors
or comets travel at about 30 km/sec. Given the relative youth of
the universe and the galaxy spontaneous life appears to be much
more likely than life propagated through random processes.
- As relatively heavy stars like the Sun begins to burn out
and turn into red giants, intelligent civilizations living around
these dying stars might find it attractive to colonize the planetary
system of nearby stars which are still actively generating energy
through nuclear fusion.
- An alternative possibility exits. The ascent of life may be
essential automatic and life may arise naturally on any suitable
planet. It may also turn out that evolution always produces
intelligent species of roughly comparable quality. In this case,
the life that arises naturally on any habitable planet would
be "just as good" as that of any civilization that
could migrate there. Once these concepts are understood, any
thinking civilization would simply stay at home.
- >Top The end of star formation:
Even the longest-lived stars die after trillions of years. Because
stars have finite lifetimes, galaxies can maintain their present
status only as long as they continue to manufacture new stars. How
long can a galaxy sustain normal star formation before it runs out
of raw material?
- The galaxy will deplete its gas reserves in another ten billion
years, roughly comparable to the current gas of the universe.
- Purely by coincidence, both star formation and stellar evolution
draw to a close at approximately the same cosmological decade.
- During the 13th cosmological decade, stars are shining brightly
and the universe is an energetic place. After the 14th cosmological
decade, the stars have gone out and the universe will appear
much darker to human eyes.
- 地球外生命:
- 白鳥座のデネブのような比較的重たい星を回る惑星にはエイリアンの文明は宿っていそうもない。太陽質量の10倍もある星は超新星となって崩壊するまで1,000万年位しか存在しない。
- 2つの証拠が進歩した文明が起こるには長い時間が必要なことを示している。その一つの例は、知能の進化には数十億年という年数が必要だった。
- 次には、他の文明が存在する兆候が全く見られないことである。特に地球外の文明から我々への接触は全くない。
- もし技術文明が短い期間で簡単に起こるのだれば、比較的近い星のいくつから検知できる信号が送られてくるのを期待ができよう。
- 相当な自信をもって、我々は銀河には約10億の棲める惑星が存在すると予測できる。この地球上の生命の歴史を見ると、一旦生命の進化が始まると、複雑性、知能、さらには技術の進歩がそれなりに起きそうなことを示している。我々は、棲める惑星の1万分の1が生命を発達させると推測している。技術的な能力を有する文明は、簡単には自滅しないと仮定して、銀河の中の文明の総数はほぼ千個と予測している。
- 星の間の距離による孤立状態は気が遠くなるほどだ。最も近い知的文明が3,000光年だけ離れていると仮定してみる。現在の近くの星に関する調整は15光年だけ終わったに過ぎない。
- 我々の銀河には千もの文明が存在しているかもしれないが、彼らとの接触を図るには大変な幸運が必要となる。
- 銀河の植民地化:
銀河の長さをランダムウォークで旅行するのに必要な時間を予測すると、銀河の直径は3万光年もあるので、生命を運ぶ隕石が動いていく距離は、星の間の典型的な距離である数光年になる。隕石や彗星は秒速30kmで移動する。宇宙と銀河が相対的には若いことを考えると、自発的な生命の方が、ランダムな仮定で伝搬する生命より大いにあり得る。
- 太陽のように相対的に重い星が燃え尽きて赤色巨星に変わっていくので、こうした死にゆく星の周辺の知識文明は、まだ核融合で活発にエネルギーを生成している星の惑星系を植民地化する魅力を感じるかも知れない。
- これとは別の可能性もある。生命の誕生は本質的に自動的で、生命はあらゆる適した惑星上で自然に発生するだろう。またほぼ同じような能力の知的な種を生み出すだろう。その場合、すべての棲める惑星上で発生した生命は、そこに移住可能な文明と大して違わない状態になるだろう。こうした概念が理解されると、すべての思慮ある文明はそのままその故郷に留まるだろう。
- 星の形成が終わる時:
最も長く生きる星でさえ数兆年後には死滅する。星の寿命には限りがあるので、銀河は新しい星を作り続けている間だけ、現在の状態を保つことができる。銀河は、原料を使い果たすまでに、どれはど長く正常な星の形成を続けていくことができるのだろうか?
- 宇宙にある現在のガスに匹敵する量の、ガスの蓄えを銀河が枯渇させるまでになお百億年かかる。
- 全く偶然だが、星の形成と星の進化とはほぼ同じ宇宙年に終わりに近づく。
- 第13宇宙年の間、星々は明るく輝き、宇宙は活気あふれる場所だった。第14宇宙年になると、星々の明かりは消えてゆき、宇宙は人間の目にずっと暗くなってしまう。
>Top 3. (15<η<39): The Degenerate Era:
- What happens when the stars have stopped shining? As the universe
enters the Degenerate Era, the effects of change are quite evident.
Conventional hydrogen-burning stars have given way to stellar remnants;
brown dwarfs, white dwarfs, neutron stars, and black holes.
- Relative number of stars:
The
left pie chart shows the relative number of stars born in different
ranges of mass.
- Brown dwarfs: 0.01 - 0.08 solar masses.
- Red dwarfs: 0.08 - 0.43 solar masses
- Sunlike stars: 0.43 - 1.2 solar masses
- Massive stars: 1.2 - 8 solar masses
- Supermassive stars: > 8 solar masses
- The right pie chart show the distribution
of stellar remnants, the objects left over after stellar evolution
has run its course.
- The brown dwarfs remain as brown dwarfs.
- But most stars (< 8 solar masses)
end their lives as white dwarfs.
- Only tiny fraction of stars (> 8
solar masses) can become black holes and neutron stars.
- >Top Brown dwarfs:
Brown dwarfs are larger than planets, smaller than regular
stars, and represent the lightest type of degenerate remnant. They
are stellar failures in the sense that they are unable to achieve
nuclear ignition of hydrogen in their interiors.
- Small stars must contract more drastically in order to achieve
a central temperature of 10 million degrees K. But, if the central
density becomes too large, degeneracy pressure dominates over
ordinary thermal pressure and supports the star before the temperature
reaches the requisite ten million degrees.
- Because they don't actually do anything, these substellar
slackers retain precisely the elemental abundance they are born
with (hydrogen).
- >Top White dwarfs:
The vast majority of stars, including our Sun, end their lives as
white dwarfs. Although a dim 0.08-solar-mass star is 100 times lighter
than a tempestuous 8-solar-mass star that shines with light of 3000
Suns, both are destined to finish their evolution by becoming white
dwarfs. The Sun will give rise to a white dwarf with about 0.6 solar
masses.
- A white dwarf with a typical mass of 0.25 solar masses has
a radius of 14,000 km. A one-solar-mass white dwarf has a radius
of only 8,700 km. More massive objects are smaller, because
their matter is degenerate. This odd behavior is in direct contrast
to ordinary matter.
- A stellarlike object thus has a minimum mass required in order
to be degenerate. This mass is approximately 1/1,000 of the
mass of the Sun, about the same mass as Jupiter.
- >Top Neutron stars:
Although white dwarfs are incredibly dense, a neutron star embodies
an even more compact form of stellar matter.
- The typical density of a white dwarf is only about 1 million
times the density of water. The nuclei of atoms are much denser,
about a quadrillion (1015) times denser than water, or a billion
times denser than a white dwarf. The neutron star is very much
like a single gigantic atomic nucleus.
- Within a highly evolved star, the central region becomes a
degenerate iron core that collapse to initiate a supernova,
often leaving behind a neutron star as a remnant. Neutron stars
are relatively rare. Only about one out of 400 stars are born
large enough to achieve detonation and leave behind a neutron
star.
- The typical mass of a neutron star is about 1.5 times that
of the Sun. Neutron stars are rather small, only about 10km
in radius.
- >Top Black holes:
The fourth possible fate of a dying star is to end up as a black
hole. After they explode and expire, the most massive stars may
leave behind a remnant larger than the maximum mass for a neutron
star, between two and three solar masses. A sufficiently massive
stellar remnant cannot be supported by degeneracy pressure and must
collapse to become a black hole.
- Black holes are strange beasts, with gravitational fields
so strong that light itself cannot escape. Because of Einstein's
relativistic speed limit, no particles or radiation are allowed
to leave a black hole. However, because of Heisenberg's uncertainty
principle, in the very long term, black holes must eventually
surrender their tightly held masses, but not until long after
the Degenerate Era has ended.
- Black holes are incredibly compact. A black hole with the
mass of the Sun is only a couple of kilometers in radius. As
another benchmark, ab black hole the size of a baseball has
about five times the mass of Earth.
- Black holes are found at the centers of galaxies. They weigh
in anywhere from 1 million to a few billion solar masses.
3.(15<η<39):
縮退する時代:
- 星が輝きをやめたら何が起こるのか。宇宙が「縮退する時代」になると、その変化は明白になる。従来の水素を燃焼する星々は、それらの残骸である褐色矮星、白色矮星、中性子星、およびブラックホールが主役となる。
- 星の相対的な数の変化:
図の左円グラフは異なる質量の範囲で誕生した星の相対的な数を示す。
- 褐色矮星:太陽質量の0.01 - 0.08
- 赤色矮星:太陽質量の0.08 - 0.43
- 太陽級の星:太陽質量の0.43 - 1.2
- 重量星:太陽質量の1.2 - 8
- 超重量星:太陽質量の8倍以上
- 図の右円グラフは星のしかるべき進化の後の残骸、すなわち星の屍としての分布である。
- 褐色矮星はそのまま褐色矮星として残る
- 太陽質量の8倍以下のほとんどの星は白色矮星になる。
- 太陽質量の8倍以上のごく一部の星がブラックホールや中性子星になる。
- 褐色矮星:
褐色矮星は惑星より大きく、普通の星よりは小さい。縮退した残骸の中で最も軽いタイプである。それらは、内部の水素の核に点火できなかったという意味では星になり損ねた星である。
- 小さな星は、1,000万度Kの中心温度を達成するために激しく収縮しなくてはならない。もし中心密度が高くなりすぎると、縮退圧が通常の熱圧力を上回り、中心核が1,000万度に達する前に星を内部から支えてしまう。
- この怠慢な褐色矮星は何もしないので、生まれたままの豊富な元素(水素)を保持している。
- 白色矮星:
太陽を含むほとんどの星は、その生涯を白色矮星として終える。太陽の3000倍の光で輝く強烈な太陽質量8倍の星と比べて、太陽質量の8%の星は重さは1/100に過ぎないが、どちらも最後は白色矮星になる運命である。太陽はほぼ6割の重さの白色矮星に生まれ変わる。
- 太陽質量の1/4の典型的な質量をもつ白色矮星の半径は14,000kmである。太陽質量と同じ白色矮星の半径の半径は8,700kmしかない。重いと小さいのである。その物質が縮退しているからだ。この奇妙がふるまいは通常の物質と正反対である。
- 星のような単体には、縮退するための最小限の質量がある。この質量は太陽質量のおよそ1/1,000で、ほぼ木星の質量と同じである。
- 中性子星:
白色矮星は信じられないほどの高密度だが、中性子星はそれよりはるかに高密度になった星である。
- 白色矮星の典型的な密度は百万に過ぎないが、原子核はこれより遙かに高密度で、約1015倍もあり、白色矮星の十億倍も高密度である。中性子星は単一の巨大が原子核と非常によく似ている。
- 高度に進化した星の内部では、中心領域が縮退した鉄の中心核になり、それが崩壊して超新星となり、その後に残骸として中性子星が残る。
- 中性子星の典型的な質量は太陽の約1.5倍である。中性子星はむしろ小さくその直径は約10kmに過ぎない。
- ブラックホール:
死にゆく星の4番目の運命はブラックホールとしてその一生を終えることである。最も高質量の星が爆発し消滅していった後に、中性子星の最大質量より大きな残骸が残ることがある。太陽質量の2〜3倍の残骸である。十分の重い星の残骸は縮退圧では支えきれないので、潰れてブラックホールになる。
- ブラックホールは奇妙な天体である。その重力場はあまりにも大きいので光でさえ脱出できない。アインシュタインの相対論による速度の限界のために、いかなる粒子も放射もブラックホールから去ることはできない。しかし、ハイセンベルグの不確定性原理によって、非常に長期間には、ブラックホールはその堅い質量もついに手放すことになるが、それは縮退の時代が終わってからしばらくはやってこない。
- ブラックホールは信じられないほど高密度である。太陽質量のブラックホールはその半径が数kmに過ぎない。もう一つの表現をすれば、野球のボールの大きさのブラックホールは地球の5倍の質量になる。
- ブラックホールは、銀河の中心にも見つかっている。それらは太陽に百万倍から数十億倍の質量がある。
-
>Top Galaxies in collision:
- Our galaxy, the Milky Way, now contains a 100 billion shining stars.
In the Degenerate Era, the sky will appear pitch black. But the
largest galaxies, held together by the gravity of cold dead stars
and dark matter, will remain intact.
- The most imminent threat facing ordinary galaxies is not the
death of their constituent stars, but rather a disruptive collision
with another galaxy. Galaxies usually live in clusters or groups.
Clusters are held together by gravity, and each galaxy traces
its own orbit through the cluster.
- Galactic collisions will affect the universe of the relatively
near future. When galaxies collide, the stars belonging to the
two original galaxies intermingle to forma a larger, but more
disorganized, composite galaxy.
- Galactic collisions are frequently associated with powerful
burst of star formation. Large clouds of gas within the galaxies
merge during such collisions and produce new stars at prodigious
rates. The most noticeable consequence for an Earthlike planet
would be the gradual doubling of the number of stars visible
in the night sky.
- The neighboring Andromeda galaxy (M31) is presently directed
on a collision course with the Milky Way. As the two galaxies
orbit around each other, and energy is lost to dynamical friction,
a future merger becomes almost inevitable.
- Galaxies in relaxation:
As the Degenerate Era gets underway collisions and near collins
between stars become increasingly important.
- Over the course of time, many scatterings take place, and
their effects slowly accumulate. The smaller and lighter stars
tend to pick up speed and gain orbital energy, whereas the heavier
stars tend to lose their orbital energy.
- >Top When a large number of stars participate in this redistribution
of wealth, the galactic structure slowly changes in a process
of dynamical relaxation. As time goes
on, more and more stars evaporate from the dying galaxy, and
are sent hurtling into intergalactic space at speed of 300 km/sec.
- Since the stars that leave have the highest energies, the
stars left behind have less energy on average. Energy is thus
drained away. In response to the mounting energy crisis, the
galaxy is compelled to grow smaller and denser.
- An unpleasant prospect awaits the low-energy stars that sink
to the galactic center, where nearly every galaxy is thought
to harbor a supermassive black hole.
- Galaxies will persist for billion of times longer than he
present age of the universe. During the next 19 to 20 cosmological
decades, most of the dead stars in a galaxy will escape through
the process of stellar evaporation. After this process of dynamical
relaxation has run its course, the life of a galaxy effectively
comes to an end.
- Long-term future of planets:
During the near term, planets will be pummeled by comets and asteroids,
which cause global climatic changes and general cataclysmic destruction.
Afterwards, the inner planets will be scorched and sterilized when
heir parent stars swell to red giant proportions. Any surviving
planets will then be forcibly removed from their solar systems and
sent off alone into the darkness of interstellar space.
- Close encounter:
This diagram shows the response of two stars to a close encounter.
After the interaction, each star has a new direction, energy, and
hence velocity. A very large number of these encounters will dynamically
relax the galaxy and thereby change its structure over vast expansions
of time.
- Degenerate stellar collisions:
As the universe grows older than several hundred billion years,
collusion will begin to add up. During the 15th cosmological decade,
hundreds to thousand of stellar collisions will rock the galaxy.
- The collisions between two brown dwarfs are of immediate astronomical,
geological and perhaps even biological interest. If its combined
mass is above the threshold for stardom, the interaction product
can contract and heat up until sustained hydrogen fusion lights
up the newly formed stellar core. The star will turn on.
- A collision between two of the typical white dwarfs produces
a somewhat large stellar object made of helium. If the final
collision product has a mass greater than 0.3 solar masses,
the object can in principle ignite helium in its interior. These
stars live for only a few hundred million years, but a mere
blink of an eye compared to their long formation time.
- If the collision product a heavier than 0.9 solar massed,
but smaller than the Chandrasekahar mass, the new object can,
in principle, sustain carbon fusion in its central core. These
brightly burring candles only last for a million years.
-
衝突する銀河:
- 我々の銀河には、現在10億の輝く星がある。縮退の時代には、空は真っ黒に見えるだろう。しかし死んだ星と暗黒物質の重力によって結びつけられた最も大きな銀河はそのまま残るだろう。
- 普通の銀河の直面する脅威は、その構成員である星の死ではなく、むしろ他の銀河との破壊的な衝突である。銀河は通常銀河団
(クラスター)を形成している。銀河団は重力で結びつけられており、それぞれの銀河は、銀河団の中で固有の軌道を描いている。
- 銀河の衝突は、しばしば星形成の激しい爆発を伴う。銀河内部のガスの大きな雲はこの衝突の際に合流し、驚異的な速さで新しい星を作り出す。地球のような惑星にいて、目立つ影響としては、夜空に見える星の数が徐々に倍増していくことだろう。
- 隣のアンドロメダ銀河(M31)が、今のところ天の川との衝突コースを進んでいる。2つの銀河は互いの周りを回っておりエネルギーが力学的な摩擦で失われているので、将来の合併は避けられない。
- リラックス (力学的緩和) する銀河:
縮退の時代が進行すると、星同士の衝突やニアミスが一層重要になってくる。
- 時間の経過につれて、多くの散乱が起き、その影響が次第に蓄積する。小さくて軽い星は速度を上げ、軌道エネルギーを獲得する傾向がある。逆に重たい星は自らの軌道エネルギーを失う傾向がある。
- 多くの星がこの富の再分配に関わってくると、銀河の構造は徐々に力学的緩和 (リラクゼーション)
の過程でゆっくり変化する。さらに時間が過ぎて、もっと多くの星が死にゆく銀河から蒸発するように、時速300kmで、銀河間の宇宙空間へ放り出される。
- 離れていく星は、最も高いエネルギーをもっているので、後に残された星には平均的により少ないエネルギーしか残らない。こうしてエネルギーは徐々に失われる。高まるエネルギー危機に対応して、銀河は小さくなり密度が高くならざるを得なくなる。
- 低エネルギーの星にとっては銀河中心に落ち込んでいくという不愉快な予測が待ち受けている。そこには超高質量のブラックホールが潜んでいる。
- 銀河は、現在の宇宙年齢より数十億倍も長く存在する。第19から20宇宙年の間に、銀河の死んだ星の大部分は蒸発過程を通して脱出するだろう。この力学的緩和のプロセスが進行すると、銀河の寿命は事実上終焉に至る。
- 惑星の長期的な未来:
近い未来には、惑星は彗星や小惑星に衝突され、全体的ない気候変化と地殻変動が起きるだろう。その後、内惑星は親星が赤色巨星へと膨張すると、焦がされ根絶やしにされるだろう。続いてあらゆる生き残りの惑星も、属していた太陽系から強制的に排除され、星の間の暗黒の空間に単独で追いやられる。
- 星の接近:
左図は2つの星が近くに遭遇した時の反応を示す。相互作用の後、それぞれの星は新たな方向、エネルギー、速度を得る。このような遭遇のほとんどの場合、銀河を力学的に緩和させ、非常に長い時間をかけてその構造を変化させる。
- 縮退した星の衝突:
宇宙が数千億年以上も経つと、衝突が増え始める。第15宇宙年の間に何百、何千という星の衝突が銀河を揺るがすだろう。
- 2つの褐色矮星同士の衝突は天文学的、地質学的、そしておそらく生物学的な関心事である。まして複合した星の合計質量がスターダムにのしあがるのに十分であれば、新しい星は収縮し、加熱、新たに形成された星の中心核で水素融合が持続的に起こる。星が誕生するのだ。
- 2つの典型的な白色矮星の衝突は、やや大きいヘリウムで作られた星状の天体が生まれる。最後の衝突で作られた天体が太陽質量の3割より大きければ、その天体は内部のヘリウムに点火することができる。このような星は数億年しか存続しない。その長い形成時間に比べれば一瞬の瞬きに過ぎない。
- もし衝突の生成物が太陽質量の9割より重く、チャンドラセカール質量より軽い場合は、新しい天体はその中心核で炭素の融合を持続できる。この明るく燃えるロウソクはわずか百万年しか続かない。
-
>Top Annihilation of dark matter:
- Galactic halos are composed largely of dark matter
(nonbaryonic particles) One of the leading candidates for the dark
matter has been named weakly interacting massive particles. These
rather strange particles, weighing in at 10 -100 times the mass
of the proton, interact only through the weak nuclear force and
gravity. They have no electric charge. They also have no interacts
via the strong force, and thus cannot be bound into nuclei.
- Since dark matter accounts for a large fraction of the mass
budget of the universe, the annihilation products from dark
matter interactions provide an important part of the inventory
of the universe at late times (20-40th cosmological decades)
The residual products from direct annihilation events in galactic
halos provide a rich variety of particles; photons, neutrinos,
electrons, positrons, protons, and antiprotons.
- Dark matter is captured by stellar remnants such as white
dwarfs. The dark matter of the galactic halo provides a background
sea of particles that are continually streaming through space.
- Over vast expanses of time, the population of these dark matter
particles gradually builds up inside the object. In the long
run, the star reaches a steady state in which annihilation occurs
within the stellar remnant at the same rate that particles are
captured form the galactic halo.
- The total power produced by a single white dwarf through this
annihilation process is about 1015 watts; power output is about
1011 times smaller than that of the Sun. Such energy generation
can continue as long as the galactic halo remains intact (20th
cosmological decades).
- Life in white dwarf atmospheres:
The scenario for life in a white dwarf atmosphere is vaguely similar
to life on Earth.
- A white dwarf is about the same radial size as Earth. Just
as life on Earth is confined near the planetary surface, any
possible life in a white dwarf atmosphere is confined to the
outer layers of the star.
- In the white dwarf, the energy source is a radiation field
heating the surface layers from below, whereas Earth is heated
from above by light from the Sun. Earth is based on liquid water.
In the white dwarf setting, chemical reactions of some type
will take place.
- The first requirement for life is a proper mix of elements.
White dwarfs of higher mass naturally contain large amounts
of carbon and oxygen.
- A white dwarf has a surface temperature of approximately 63
degrees K. At the outer stellar surface, the photons have an
average energy of a small fraction of an electron volt.
- A white dwarf powered by the annihilation of dark matter generates
energy at a rate of 1015 watts. (=1% of the total power accessible
to Earth's biosphere)
- For lower temperatures, the effective pace of life is slower,
and it takes longer for a creature to experience the same number
of instants of consciousness. Compared to life on earth, life
in white dwarf atmospheres thus lose a factor of 5 for having
a slower metabolic rate and loses another factor of 100 for
having less power. This loss of a factor of 500 is more than
made up by the time available, which is 100 billion times longer.
We estimate that life in a white dwarf atmosphere has a numerical
advantage is 100 million times.
- We have no general guarantee that time, energy, and chemistry
are sufficient for biological emergence.
- >Top Proton Decay:
One of the surprises coming out of particle physics
in 20th century is that the proton will not live forever.
- Many theories of physics forbids the decay of protons. This
law can be concisely stated; baryon number is conserved. Each
proton or neutron contains one unit of baryon number. Particles
like electron, positron have zero baryon number, as do photons.
So if a proton decays into a positron, some baryon number is
lost in the process.
- The newer versions of particle theories; protons will decay
over extremely long spans of time, much longer than the current
age of the universe. The time required for proton decay is about
30 cosmological decades.
- To detect the decay process, one needs a large number of particles.
If we get a large box containing 1032 protons (20m x 5m x 2m
pool), then one proton per year would decay within the experimental
apparatus. We don't need to wait 1032 years to find out the
answer.
- In the early universe, some process involving baryon number
violation produced the matter that we see in the universe today.
- The quarks inside a proton do not sit around at rest. If the
quarks are close enough together, they can coalesce into a microscopic
black hole. This process is poorly understood.
- One of the Proton Decay:
One
of the possible channels for proton decay is depicted here. In this
case, the end result of the proton decay is a positron and neutral
pion. The pion is highly unstable and quickly decays into radiation
(ie, photons). If the decay occurs in a dense environment like a
white dwarf, the positron will quickly annihilate with an electron,
producing two more high-energy photons.
- Ultimate fate of degenerate remnants:
The final chapter of stellar evolution plays itself out as the protons
decay. We adopt a representative proton lifetime of 37 cosmological
decades.
- During the evolutionary phase when protons decay, the chemical
makeup of a white dwarf changes completely. The original carbon
nuclei are reduced to a single particle and the star ends its
life as pure hydrogen.
- As a white dwarf continues to lose mass through proton decay,
its structure changes markedly. A white dwarf expand in radial
size as it withers away. The star consists of a frozen array
of hydrogen atoms - a large ball of hydrogen ice.
- The neutron stars evaporate in a similar fashion. During the
37th cosmological decades, neutron stars softly glowing at 3
degrees K will be among the hottest objects in the universe.
When the neutrons are no longer degenerate, they transform themselves
into protons, electrons, and antineutrons.
- By the 40th cosmological decade, nearly all of the protons
in the universe have decayed and the degenerate stellar remnants
are gone. The seemingly resolute and indestructible stellar
remnants have given way to a diffuse sea of radiation, mostly
photons and neutrinos, with a small admixture of positrons and
electrons.
-
暗黒物質の消滅:
- 銀河のハロ-はほとんど暗黒物質 (非バリオン粒子) からなる。暗黒物質の筆頭候補の一つは、弱い相互作用をする質量のある粒子である。この奇妙な粒子は、弱い核力と重力だけに反応し、陽子の10〜100倍の質量がある。これらには電荷がなく、強い核力と反応しないので、原子核の中に結合されることはない。
- 暗黒物質は宇宙の質量の中の大部分を占めるので、暗黒物質の相互作業による消滅の派生物は、後の段階で宇宙の在庫にとって重要となる。(第20〜40宇宙年)
銀河ハローでの直接的な消滅現象による派生物には、光子、ニュートリノ、電子、陽電子、陽子、反陽子など多彩な粒子が生まれる。
- 暗黒物質は白色矮星のような星の残骸によって捕獲される。銀河ハローの暗黒物質は背景の海となり、常に宇宙空間を流れる。
- 悠久の時間をかけて、これらの暗黒物質の粒子は徐々にこれらの物体の内部に集積してくる。長期的には、それらの粒子は星の屍内部での消滅と銀河ハローからの捕獲とで安定した状態に達する。
- 一つの白色矮星がこの消滅過程によって得る全パワーは1015ワットであり、これは太陽の1011分の1のパワー出力である。このようなエネルギー生成は銀河ハローがある限り第20宇宙年も続く。
- 白色矮星の大気圏内生命:
白色矮星の大気圏内での生命のシナリオはおよそ地球上の生命に似ている。
- 白色矮星は、ぼぼ地球と同じサイズである。地球上の生命が惑星表面に限られているように、白色矮星に生命があるとしたらその星の外部の層に限定される。
- 白色矮星では、エネルギー源は地下から表面の層を暖められる放射場である。地球では太陽の光によって上から暖められる。地球では水が基盤だが、白色矮星では、何らかの化学反応が起きる。
- 生命の最初の必要条件は元素がほどよく混合していることである。高質量の白色矮星は大量の炭素と酸素を含む。
- 白色矮星の表面温度は約63度Kである。星の外部層では光子のエネルギーレベルは1電子ボルトに満たない。
- 白色矮星は暗黒物質の消滅によって1015ワットのパワーを得ており、これは地球の生物圏が利用できる全パワーの1%に相当する。
- 低温では生命の実質的なペースは遅くなり、生物が同じ数の意識の瞬間を経験するのにはより長い時間がかかる。地球上の生命に比べて、白色矮星の大気中の生命はより緩慢な代謝率をもっているので5倍も損をし、かつ低パワーしか利用できないので100倍損をする。この500倍の損失も利用可能な時間が1,000億倍も長いことで埋め合わせられる。結局、白色矮星の大気中の生命は数字的には約1億倍も有利な条件にあると推測できる。
- 但し時間とエネルギーと化学反応があれば生命が出現する保証はされていない。
- 陽子崩壊:
20世紀の素粒子物理学がもたらした驚きの一つは、陽子は永久には存続しないということである。
- 物理学の多くの理論は、陽子は崩壊しないとしている。この法則は簡潔に表現するれば、「バリオン数は保存される」というものである。陽子や中性子はぞれぞれ1つのバリオン数をもつ。電子や陽電子は、光子と同様にバリオン数はゼロである。従った陽子が崩壊して陽電子になるとその過程でバリオン数は失われる。
- 新しい素粒子理論によれば、陽子は、現在の宇宙の年齢より遙かに長いような時間で崩壊するだろう。この陽子崩壊に必要な時間は約30宇宙年である。
- 崩壊過程を検知するには、大量の粒子を必要とする。1032個の粒子を容器 (20m x 5m x 2m のプール)
を考えると年に1個の陽子が実験装置内で崩壊するだろう。我々はその答えを見つけるために1032年も待つ必要はない。
- 初期宇宙では、バリオン数が破れて現在の宇宙に見られる物質を生成した。
- 陽子内のクォークはその場所に留まっているのではない。もしクォークがあまりにも接近しすぎると、合体してミクロのブラックホールになる。但しこの過程はよくわかっていない。
- 陽子崩壊の一例:
左図は陽子崩壊の過程の一つを描いている。陽子崩壊の結果、陽電子と中性パイ中間子が生成される。パイ中間子は非常に不安定なのですぐに放射線
(光子) に変わる。もしこの崩壊が白色矮星のような高密度の環境で起こると、陽電子はすぐに電子と衝突して消滅し、2つのさらに高エネルギーの光子を生成するだろう。
- 縮退した残骸の究極の運命:
星の進化の最終章は陽子の崩壊で終わる。ここでは陽子の寿命として典型的な第37宇宙年を採用する。
- 陽子が崩壊する進化の段階で、白色矮星の化学構造は一変する。元の炭素の原子核は単一の粒子となり星はその生涯を純粋な水素となって終わることになる。
- 白色矮星が陽子崩壊によって質量を失い続けるに従ってその構造は著しく変化する。白色矮星は衰退するに従ってその半径は膨らんでいく。その星は凍った水素原子で構成され、巨大な水素の氷となる。
- 中性子星も同様に蒸発する。第37宇宙年の間に中性子星は徐々に3度Kとなるが、その宇宙でも最も熱い物体の中に入る。中性子星がもはや縮退しなくなると、陽子と電子と反ニュートリノになる。
- 第40宇宙年になると宇宙にあるほとんどの陽子は崩壊し、縮退した星の屍は消えてゆく。一見堅固で崩壊しないと思われていた星の屍も、おもに光子とニュートリノ、それに陽電子と電子が少し混ざった放射線だけの海にとって代わる。
>Top 4. (40<η<100):
The Black Hole Era
- When the universe reached its 40th cosmological decade, the black
holes reign supreme. They provide the light, the heat, and the dynamics
to keep thins interesting.
- >Top Schwarzschild radius:
At a well-defined radius, the escape speed finally exceeds the
speed of light. This point of no return mark the location of
a effective surface for the black hole.The Schwarzschild radius
of a one-solar-mass black hole is a few km. A-million-solar-mass
black hole has a radius of 3 million km, about 4 times large
than the present-day Sun. If Earth were compacted down to form
a black hole, it would be about the size of a marble. Since
no information can travel out of this local region, it is effectively
separated from the rest of the universe. (event horizon)
- Independent of the production mechanism, once a particular
black hole has formed, its characteristics are completely described
by only three : the mass, electric charge, and rotation rate
(angular momentum). A black hole has no hair. Except for these
3 defining quantities, nothing remains of a black hole's history.
A relative nearby black hole candidate, Cygnus X-1, has a mass
about 10 times that of the Sun, and a radium of roughly 30km.
- Supermassive black hole which anchors the
core of a galaxy: billion of solar masses, and have Schwarzschild
radii larger than the size of Jupiter's orbit. Quasars:
as gas funnels into the central black hole, friction heats up
the disk and powers a brightly shining quasar.
- Primordial black holes: they must have been
created shortly after the big bang. That are also likely to
be very small, much less massive than stars.
- In spite of their inviolable reputation, black holes will
not last forever. Larger, more massive black holes live much
longer than smaller ones.
- Basic black hole weirdness:
In the Black hole era, general relativity and black holes reign
supreme.
- Einstein realized that the equivalence between gravity and
acceleration is extraordinarily profound. (Statement of equivalence)
- Another far-out possibility is to place an observer near a
black hole in an orbit subject to extreme time dilation. The
observer could then effectively "travel" into the
future.
- Black holes distort the structure of space just as severely
as they warp the passage of time.
- Near a black hole, the local curvature of space is so strong
that the distance to the center of the black hole is infinitely
longer than the distance around the circumference. The curvature
creates the event horizon, the curvature causes the extreme
differences in the passage of time for different observers,
and the curvature bestows on large black holes the fortitude
to last into the extremely distant future.
- By the 40th cosmological decade, the galaxies are completely
destroyed. The sole legacy of each galaxy is one supermassive
black hole and about one million stellar black holes. The horizon
is 1030 times farther away than it is now. If the large scale
geometry of space-time is flat, the observable universe will
contain about 1040 black holes with supermassive status, and
nearly 1046 stellar black holes.
- Gravitational radiation:
As the Black Hole Era progresses, energy is slowly drained out of
orbiting gastronomical systems due to the dissipative effects of
gravitational radiation.
- Because gravity is so weak, the amount of energy carried in
gravitational waves is generally very small.
- Hawking radiation and decay of black holes:
Black holes are not completely black. Over vast expanses of time,
they radiate heat into space at an extraordinarily slow rate. So
an object that generates heat must also be slowly losing mass.
- Black hole evaporation is one of the few calculable results
that fall in the realm of quantum gravity. The quantum
mechanical process that causes black hole evaporation was discovered
by Stephen Hawking in 1974.
- Since black holes radiate heat (energy), they must also have
a temperature. Every black hole is slightly hotter than absolute
zero. A black hole with the mass of Sun has only 10−7 degrees
K. Larger black holes hold onto their mass energy more tightly,
radiate much less efficiently, and have even lower temperature.
- The universe is immersed in a bath of radiation left just
after the big bang. (about 3 degrees K)
- If the universe is flat, the temperature of the sky becomes
colder than the temperature of a one-solar-mass back hole during
the 21st cosmological decade. Similarly, black holes with one
billion solar masses begin to evaporate during the 35th cosmological
decade.
- Inside black holes:
What is actually inside a black hole?
- Roger Penrose proved a theorem which shows that every black
hole must contain a singularity. While quantum
gravity undoubtedly determines the true nature of the singularity
within a black hole, a definitive description is currently unavailable.
- Life in the black hole era:
- The metabolic rate of an abstract creature is proportional to
its operating temperature. This slowing down of metabolic rates
and thought processes is more than compensated by the huge increase
in the amount of available time.
- A human being has an effective complexity value of 1023. To
obtain this value, we use the power output of about 200 watts
for a person operation at a temperature of about 300 degrees K.
Suppose that we consider a stellar black hole to be a living creature.
It would have an effective complexity value of only about 1013.
- The final moments:
A black hole's last seconds are dramatic.
- As a black hole shrinks in mass and size, its temperature
and evaporation rate gradually increase. After shedding 95%
more of its mass, the black hole surface is as hot as the Sun.
It persists for 1032 years. During most of this span, the evaporating
hole primarily emits massless particles such as neutrinos and
photons.
- As the end draws near, the black hole becomes blindingly bright,
and the final vanishing point arrives with a certifiable explosion.
As it expires, the black hole produced more than just massless
particles including electrons, positron, protons, and antiprotons.
4. (40<η<100):
ブラックホールの時代
- 第40宇宙年になることにはブラックホールが君臨している。それらは、光、熱、そして物事をおもしろくする動力学を与えてくれる。
- シュヴァルツシルド半径:
明確に定義された半径では、脱出速度が光速を超える。この後戻りできなくなる地点が、ブラックホールの表面になっている。太陽質量の大きなのブラックホールの半径
(シュヴァルツシルド半径)は数kmである。太陽質量の百万倍のブラックホールの半径は300万kmで現在の太陽の約4倍である。もし地球が圧縮されてブラックホールになったとすれば、ビー玉のサイズになるだろう。この局所的な領域からはどんな情報も伝わってこない。それは事実上宇宙の他の部分から切り離されている。(事象の地平線)
- 生成メカニズムにかかわらず、一旦ブラックホールが形成されると、その特徴は質量・電荷・角運動量の3つの量によって完全に記述される。ブラックホールには毛がない。この3つの量以外にブラックホールの歴史を示すものは何も残らない。比較的近くのブラックホール候補は白鳥座のX-1星であり、それは太陽質量の10倍で半径は約10kmである。
- 超高質量ブラックホールは銀河の中心に錨のように存在しており、それは太陽質量の数十億倍もあり、シヴァルツシルド半径は木星軌道よりも大きい。クェイサーは、中心のブラックホールに向かってガスが集められる際の摩擦によって円盤が熱せられ非常に明るいクェイサーにエネルギーが与えられている。
- 原始のブラックホールは、ビッグバンの直後に作られてものに違いない。それらは非常に小さく星よりもずっと軽いに違いない。
- その犯しがたい名声にもかかわらずブラックホールも永久には存続しない。より大きくて重たいブラックホールは小さいものよりもずっと長生きする。
- ブラックホールの奇妙な点:
ブラックホールの時代になると、一般相対性理論とブラックホールが支配的になる。
- アインシュタインは重力と加速度の等価が極めて重要であることに気づいていた。(等価原理)
- もう一通の常識はずれの可能性は、観測者をブラックホール近くの極度に時間の間延びを受ける軌道に乗せることであろう。観測者は事実上未来へ旅することができる。
- ブラックホールは、時の経過を歪めるのとおなじように、宇宙空間の構造も歪ませる。
- ブラックホールに近くでは空間の局所的な曲がりがあまりにも大きいのでブラックホールの中心までの距離はその周辺より無限に長くなる。その曲がり具合は事象の地平線を作り、異なる観測者に対する時の刻みに極端な違いを生じさせる。そしてその歪み具合が大きなブラックホールに遙かな未来まで存続するのに耐える力を与える。
- 第40宇宙年までに銀河が完全に崩壊してしまい、それぞれの銀河の唯一の遺産は一つの超高質量ブラックホールと約百万個の星のブラックホールである。地平線は現在よりも1030倍も遠くにまで拡がっている。もし時空間が幾何学的に平らであるならば、観測可能な宇宙は1040の超高質量級のブラックホールと1046個の星のブラックホールを含んでいる。
- 重力放射:
ブラックホールの時代の進行につれて、重力放射がもたらす散逸効果によって、軌道を描く天文的な系からエネルギーがゆっくりと枯渇していく。
- 重力は非常に弱いので、重力波が運ぶエネルギーの量は非常に小さい。
- ホーキング放射とブラックホールの崩壊:
ブラックホールは完全には黒くはない。悠久の時間のい間にブラックホールは、極めてゆっくりした速度で宇宙空間に熱を放射する。熱を生ずる物体は、また徐々に質量を失っていく。
- ブラックホールの蒸発は量子重力の領域の計算によって予測できる結果の一つである。ブラックホールに蒸発を引き起こす量子力学的なプロセスは1974年にスティーブン・ホーキングによって発見された。
- ブラックホールは熱 (エネルギー)を放出する。それらには温度があるに違いない。どのブラックホールも絶対零度よりほんの少し熱い。太陽質量のブラックホールの温度は10−7度Kである。大きなブラックホールはより強固に質量を保持し、熱放射の効率は劣るのでより低温を保っている。
- 宇宙はビッグバン直後から残された放射の浴槽に浸かっている。(約3度K)
- もし宇宙が平坦ならば、第21宇宙年には、空の温度は太陽質量と同じブラックホールの温度より低くなる。同様に第35宇宙年には太陽質量の百万倍のブラックホールは蒸発し始める。
- ブラックホールの内側:
ブラックホールの内側はどうなっているのか。
- ロジャー・ペンローズは「すべてのブラックホールには特異点がある」という定理を証明した。量子力学は間違いなくブラックホールの特異点の真の性質を決めているが、決定的な記述は今のところ入手できない。
- ブラックホール時代の生命:
- 抽象的な生命の退社率はその稼働する温度に異例する。代謝率や意識のプロセスはそれが活用できる時間が圧倒的に延びることで補える。
- 人間は1023という実質的な複雑性をもっている。この値を得るために、我々は一人当たり200ワットのパワーを300度Kで利用している。星のブラックホールを生き物と見なしたとしても、それは1013の複雑性の価値しかもっていない。
- 最後の瞬間:
ブラックホールの最後の数秒は劇的である。
- ブラックホールが質量と大きさを減らして行くにつれて、その温度も蒸発率も徐々に増加する。その質量の95%を失うと、ブラックホールの表面温度は太陽と同じになる。それは1032年間も存在し続け、その間蒸発していくブラックホールは、ニュートリノや光子のような重さのない粒子を放出する。
- 終わりが近づくにつれて、ブラックホールはまぶしく輝き、最後の消えゆく段階には爆発を伴う。消えゆくにつれて、ブラックホールは重さのない粒子以外の電子、陽電子、陽子、反陽子を放出する。
>Top 5. Black apocalypse:
- Let's consider the rather dismal scenario in which Earth collides
with a black hole.
- The intruding black hole formed during the death of a massive
star in the disk of our galaxy. It rushes toward Earth with
a speed of several km/sec. The first indirect hints of the encroaching
black hole come millennia before the actual collision.
- As the tiny behemoth passes through the Oort cloud, it dislodges
comets from their orbits. Some are thrown into deep space, large
numbers are enlisted into orbit around the back hole.
- Centuries before the black hole's arrival, astronomers notice
sizable changes in the orbits of the outer planets as they respond
to the black hole's gravitational influence.
- A star of two solar masses charging through the outer reaches
of of the Oort clouds would appear as bright as a streetlight
a few blocks away.
- Viewed from afar, a black hole acts like a lens because its
gravity warps space-time so as to magnify and distort the images
of objects lying along the line of sight.
- As the black hole crosses the orbit of Pluto, the planets
engage in wild deviations from their normal orderly courses.
Jupiter and Uranus are captured by the black hole, while Saturn
and Neptune are flung out to uncharted realms of interstellar
space.
- From Earth's unfortunate vantage point, the black hole approached
from the opposite side of the Sun. The combined gravity of the
Sun and the black hole pull the earth through a distorted echo
of an ordinary year. Now, over the course of a few final days,
all that orderly clockwork is ruined.
- The end falls quickly. Observed through a telescope, the black
hole appears like a psychedelic comet, surrounded by faintly
glowing gas and warped images of background stars and galaxies.
As the tidal forces of the black hole take hold, the side of
Earth facing the back hole is pulled with greater force. Stresses
mount in the crust and earthquakes rock the surface. Tsunamis
wash over the continents. The planetary crust rips apart along
old fault lines. Earth is pulled like taffy into a disk of vaporized
rock which forms a whirlpool in its stampede to enter the impinging
black hole. The energy released during the final demise is visible
far beyond the galaxy.
5.ブラックホールに呑まれるとき:
- 地球がブラックホールと衝突する惨めなシナリオを想定してみよう。
- 侵入してくるブラックホールは、我々の銀河の中の重たい星の死から生じたものである。それは地球に向かって秒速数kmの速度で突進してくる。侵入してくるブラックホールの最初の兆候は実際の衝突の千年も前から間接的な兆しが見られる。
- 小さいグロテスクな怪獣がオールトの雲を通り過ぎると、彗星群を軌道から追い払う。一部は深宇宙へ投げ出され、多くはブラックホールの軌道に引き寄せられる。
- ブラックホールの到着する何世紀も前から天文学者は外惑星がブラックホールの引力の影響をうけて軌道に大きな変化が生じているのに気づく。
- 太陽の2倍の質量をもつ星は、オールトの雲の外部領域に到達すると、数ブロック先の街灯のように明るく見えるだろう。
- 遠くから見るとブラックホールは重力で時空を歪めて、視線にそった天体の像を拡大し歪めて見えるレンズのように振る舞う。
- ブラックホールが冥王星の軌道を横切ると、惑星はその規則的な進路から大きくずらされる。木星と天王星はブラックホールに捕獲され、土星と海王星は星間の未知の領域へ投げ飛ばされる。
- 地球の立場からはブラックホールは太陽の反対側から近づいてくる。太陽とブラックホールの結合した引力が普通の一年の間中、地球を引っ張り続ける。今や最後の数日の内に、規則正しい時計の動きは終わりを告げる。
- 終焉は急にやってくる。望遠鏡で観察するとブラックホールはサイケ調の彗星のようであり、ぼんやりした輝くガスと、背景の星や銀河がゆがんで見える。ブラックホールの朝夕力が支配すると地球のブラックホールに面した側はより大きな力で引っ張られる。地殻の中でのひずみが高まり地震が地面を揺らす。津波が大陸を洗い流す。地殻は古い断層線によって引き裂かれる。地球は飴のように引っ張られ蒸発した岩石の盤状となり衝突するブラックホールに落ち込むように殺到して渦状を形成する。この最後の断末魔から放出されるエネルギーは銀河を越えた遠方からも見える。
>Top 6. (101<η): The Dark Era:
- At the present time, the density of protons in the universe is
about one particle per cubic meter.
- For a flat universe, which expands forever but slow down as
it does so, the universe grows by a facto of 1060 from now
until the start of the Dark Era. Future density of the positrons
is about one particle for every 10182 cubic meters.
- Consider that the entire observable universe of today has
a volume of only about 1078 cubic meters. The positron density
of the Dark Era would be about one particle within a volume
that is 110104 times larger than the universe to today.
- At the present time, the cosmic background radiation left
over from the big bang is the most energetically interesting
This radiation has 3 degrees K and characteristic wavelength
of 1-2 mm. A flat universe, grows by the huge factor of 10^60
between now and the star of the Dark era.
- The background sea of stellar radiation will eventually overwhelm
the radiation left over from the big bang during the 12th cosmological
decade. As the relatively near future unfolds, this radiation
will be predominantly produced by red dwarfs, which are the
smallest, most common, and longest-lived stars.
- Heat Death:
- Various processes to the radiation of
the universe:
The
contribution of various processes to the radiation background of
the universe are shown here as a function of time, for cosmological
decades 5 to 90. The vertical axis presents the relative energy
in radiation from several sources; radiation left over from the
primordial universe, starlight, dark matter annihilation, proton
decay, and black hole evaporation.
- >Top Heat Death:
A continually expanding universe never reaches true thermodynamic
equilibrium because it never reaches a constant temperature.
- The background temperature of the universe continues to decrease
because of the expansion. However, the expanding universe can
become purely adiabatic; still become a dull and lifeless place
with no ability to do physical work. (Cosmological heat death)
- Life and death of positronium:
Perhaps the most lively feature of the Dark Era will be processes
involving positronium atoms.
- With no protons or neutrons left, conventional atoms become
impossible constructions. Electrons and positrons can be cast
into atomic structures that are analogous to conventional hydrogen
atoms. (known as positronium)
- the typical size of positronium forged during the Dark Era
is trillions of light-years, larger than the entire observable
universe of today. The electron and the positron slowly circle
each other and gradually give up extraordinarily small amounts
of radiation as their orbits become ever smaller.
- With their enormous starting sizes, the positronium atoms
take a rather long time to decay, about 145 cosmological decades.
- Never-ending annihilation:
The annihilation rate slows down markedly as the universe expands,
but particle annihilation continues as long as the universe exists.
- This conservation practice underscores the energy crisis of
the future and defines exactly what it means for the universe
to slow down as it gets older.
- Paul davies has described this late phase of evolution of
the universe as an "eternal death." This ever dying
universe continues to evolve and continues to slow down, but
never reaches a final moment of deathlike closure.
- Tunneling processes and phase transitions:
- The most optimistic scenario arises when the cosmological constant
is currently positive and the universe tunnels into a new vacuum
state with exactly zero vacuum energy. In this fortuitous case,
the new universe, with its new physical laws, has a chance to
develop new stars for complexity and perhaps even new starts for
life.
- On the other hand, if the cosmological constant of is already
exactly zero and the new vacuum state has a negative value,
then a far more serious apocalypse takes place.
- A future phase transition sweeping over the
entire universe through the action of quantum tunneling is one
of the more speculative topics. Nevertheless, the act of tunneling
from a false vacuum into a true vacuum changes the nature of the
universe more dramatically than just about any other physical
process.
- It is even possible for the universe spontaneously to create
new "child universe" through a quantum tunneling process.
In this case, a bubble of the higher energy false vacuum nucleates
in an otherwise empty space-time, in the true, lowest energy vacuum
state.
- The newly created bubble becomes a new and separate universe
(child universe)
- Observers living within the bubble see their local universe
in a state of exponential expansion, much like the inflationary
phase of our universe in its earliest moments.
- Observers situated outside the bubble, those left behind
in the empty space-time background of our present universe,
see the newly created universe as a collapsing black hole
that quickly become causally disconnected from our universe.
6. (101<η):
暗黒の時代:
- 現在は、宇宙の陽子の濃度は1立方mに約1個である。
- 平坦な宇宙の場合、宇宙は無限に膨張するにつれてその速度は遅くなるものの、暗黒の時代の時代が始まるまでには1060倍にまで膨張する。将来の陽電子は10182立方mに1つという密度になる。
- 今日観測可能な宇宙全体の大きさは1078立方mに過ぎない。暗黒時代の陽電子の密度は、現在の宇宙よりも10104倍もの体積の中に1個という割合になる。
- 現在、ビッグバンの残存である宇宙の背景放射は、エネルギー的に最も興味深い出来事である。この放射は3度Kでその波長は1-2mmである。平坦な宇宙では、現在と暗黒の時代とでは1060倍にも拡大するのである。
- 星の放射による背景の海は、第12宇宙年からはビッグバンの残存の放射を結果的に圧倒することになる。比較的近い未来では、この放射はもっとも小さくごぐありふれた最も長寿の星である赤色矮星によって放射されるものによって占められる。
- 宇宙放射の各プロセス(左図)
宇宙の放射の背景に、様々なプロセスが占める割合が、ここでは宇宙年5年から90年の間の時間関数として示してある。縦軸は発生源による放射のエネルギーを表す。原始宇宙から残された放射、恒星の明かり、暗黒物質の消滅、陽子崩壊、およびブラックホールの蒸発。
- 熱的死:
いつまでの膨張し続ける宇宙には決して熱力学的平衡には到達しない、それは一定温度にならないからである。
- 宇宙の背景温度は膨張するにつれて引き続き下がり続ける。膨張宇宙は断熱的な状態になり得る。この場合宇宙は物理的な仕事をする能力のない沈滞した生命のない場所になる。(宇宙論的な熱死)
- ポジトロニウムの生と死:
暗黒時代の最も活気のある出来事はポジトロニウム原子にかかわるプロセスだろう。
- 陽子も中性子もないので、従来の原子は作られない。電子と陽電子が従来の水素原子に似た原子構造を形作る。(ポジトロニウム)
- 暗黒時代に作られるポジトロニウムの典型的な大きさは数兆光年であり、現在観察可能な宇宙全体よりも大きい。電子と陽電子は互いにゆっくりと周回し非常に少量の放射をしながく徐々にその軌道を小さくしていく。
- このようなポジトロニウムは当初から異常な大きさなので崩壊するのにほぼ145宇宙年かかる。
- 終わりなき消滅:
宇宙が膨張するにつれて粒子の消滅率は際だって遅くなるが、粒子の消滅は宇宙が存在する限り続く。
- この節約のやり方は未来のエネルギー危機の特徴で、宇宙が年をとるにつれて宇宙がゆっくり変化していくことの意味の定義となっている。
- ポール・デービスは宇宙進化の最終段階を「永遠の死」と表現している。この品詞の宇宙はさらに進化とつづけ衰え続けるが決して死という最期の瞬間を迎えることはない。
- トンネル過程と相転移:
- 最も楽天的なシナリオは宇宙常数が現在は正で、宇宙が正確にゼロの真空エネルギーをもつ新しい真空状態へトンネルする場合である。この幸運な場合は、新しい物理法則に従う新しい宇宙は複雑性や新たな生命のスタートのチャンスともなり得る。
- 反面、宇宙常数がすでに正確にゼロで、新たな真空状態が負の場合はずっと厳しい破局が生じる。
- 未来の相転移は量子トンネル作用を通じて宇宙全体を掃き捨てることであり、最も推理的な話である。にもかかわらず虚の真空から真の真空へのトンネル作用は、宇宙の性格を他の物理プロセスより劇的に変えてしまう。
- 量子トンネル過程を通じて新たな「子供宇宙」を自発的に作ることさえ可能になる。この場合高エネルギーの虚の真空バブルか、あるいは空の時空で真の真空の低エネルギー状態の中に核を形成する。
- 新たに作られるバブルは新しい独立の宇宙となる (子供宇宙)
- 観測者がバブルの中にいれば、彼らの局所宇宙は、我々の宇宙の初期のインフレーションによく似たような指数関数的な膨張となる。
- 観測者がバブルの外側にいれば、現在の宇宙のは空の時空に取り残され、新たなに作られる宇宙は、崩壊しつつあるブラックホールのように我々の宇宙からすぐに因果的に切り離されていく。
Comment
- The chlonolgy of the univese is a never ending story. As we, a
living creature, have the end story, we will be attracted by such
a story.
- Furthermore the universe is full of surprise and creativity. We
must have more imagination to understand our universwe.
- 宇宙の物語はまさに終わりのない物語である。我々生物は終わりがあるからこそ、終わりのない物語に惹かれるのかも知れない。
- それと宇宙には意外性と創造性が詰まっている。我々ももっと想像力を発揮して我々の宇宙を理解する必要がある。
![]() |
The Five Ages of the Universe
|
Cat: SCIPub: 1999#: 0113b |
Fred Adams and Greg Laughlin |
01523u/18204r |
Title |
The Five ages of the Universe |
宇宙の5段階 |
---|---|---|
Author |
Fred Adams & Greg Laughlin | F.アダムス、G.ローリン |
Published |
1999 |
1999年 |
Index |
||
Tag |
; ; 75% proton; Annihilation of dark matter; Billions years for intelligence; Black hole; Brown dwarf; Civilizations 1000; Cosmological decade; Dynamic relaxation; First three minutes; Four windows; Gravity or entropy; Heat death; Homogenous & isotropic; Horizon problem; ; More massive smaller; Neutron star; Proton decay; Relative isolation; Schwartzschild radius; Sun ranks 4th; ; ; Vacuum energy; White dwarf; ; | |
Why? |
|
|
Summary |
要約 |
>Top 0. Introduction:
|
0.序:
|
|
|
>Top 1. (-50<η<5): The Primordial Era:
|
1.(-50<η<5) 原始時代:
|
|
|
>Top Quark and Antiquarks:
|
クォークと反クォーク:
|
>Top2. (6<η<14): The Stelliferous Era
|
2.(6<η<14): 星多き時代
|
|
|
|
|
|
|
>Top 3. (15<η<39): The Degenerate Era:
|
3.(15<η<39): 縮退する時代:
|
|
|
|
|
>Top 4. (40<η<100): The Black Hole Era
|
4. (40<η<100): ブラックホールの時代
|
>Top 5. Black apocalypse:
|
5.ブラックホールに呑まれるとき:
|
>Top 6. (101<η): The Dark Era:
|
6. (101<η): 暗黒の時代:
|
Comment |
|
|